Entry Version:
Citation:
Pancreapedia: Exocrine Pancreas Knowledge Base, DOI: 10.3998/panc.2013.1
Attachment | Size |
---|---|
![]() | 475.37 KB |
Gene Symbol: RAB3A, RAB3B, RAB3C, RAB3D
1. General Information
Rab proteins constitute the largest family of Ras-related small G proteins and play a role in regulating the specificity of membrane trafficking (63, 78). Because of its potential importance in acinar cell digestive enzyme secretion Rab3 is an important subject for review. Rab3 was originally identified in brain and shown to be localized to synaptic vesicles (24). Because of its localization and homology to the yeast protein Sec4, it was believed to play a role in the terminal steps of secretion. Subsequent molecular cloning revealed four members of the Rab3 family termed 3A (the form originally found in brain), 3B, 3C, and 3D. These four forms show 75-80% amino acid identity with the amino and carboxyl terminal regions being most distinct. All four forms are present in brain, but most other tissues contain one or two forms. Rab3B and 3 D have been found in exocrine, endocrine, epithelial and adipose cells (60). A single form of Rab3 exists in C. elegans and is believed to play a role in neurotransmitter release (47), while in sea urchin eggs a Rab3 is believed to play a role in cortical granule exocytosis (13). Rab3 isoforms have been identified in different tissues and cell types where it has been suggested to play a role in secretion (Table 1). Note that in some cases Rab3 inhibits and in others it stimulates secretion.
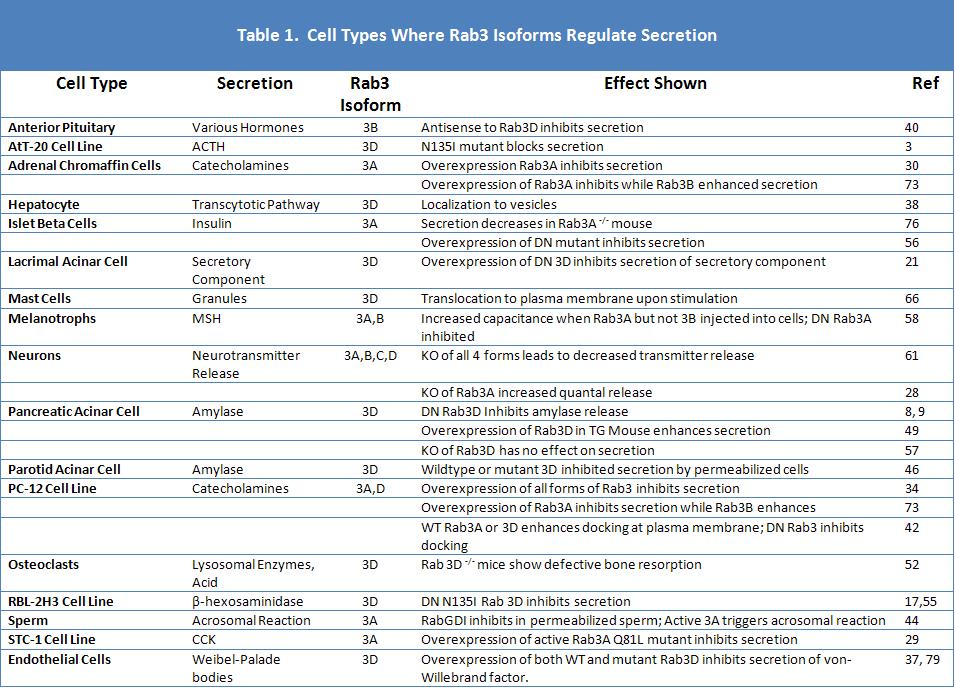
Structurally, Rab3 species contain conserved functional domains similar to other Rabs. These include four regions participating in guanine nucleotide binding, and effector region corresponding to the effector region in Ras, and a CXC prenylation motif at the C-terminal (45). The latter is used for the addition of two geranylgeranyl groups to the cysteine residues which attach the Rab3 to membranes (35). The crystal structure of Rab3A in the active configuration or bound to Rabphillin has been determined (19, 50) and Rab3D has been fit to the same model with 6 β-strands and 5 α-helices (45). The structure of the Rabs changes upon binding GTP and mutant Rab3 species have been described similar to Ras that are locked into active and inactive configurations (6). Rab3A and 3D mouse genes possess 5 exons and Rab3D has been localized to chromosome 13 (1,5).
Similar to other small G proteins, the guanine binding state of Rab3 proteins is regulated by guanine nucleotide exchange proteins or factors (GEPs or GEFs) and GTPase activating proteins (GAPs). To date, two Rab3 GEFs have been identified. Rab3 GEP was purified from brain and acted on Rab3A, 3C and 3D (72). Although it did not regulate Rab 2, Rab 5, Rab 10 or Rab 11, a recent study indicates it can act on Rab 27A and 27B (23). Rab3 GEP is identical to the human DENN/MADD protein and contains a death domain which can bind the TNFR1 (15). Rab3 GEP knockout mice die at birth and embryonic synaptic transmitter release is impaired (64). The second Rab3 GEF, known as GRAB, interacts with inositol hexakisphosphate kinase and Rab3A and its protein expression is primarily in brain (41). There are no known GEFs specific for individual Rab3 isoforms such as Rab3D. There is one known Rab3 GAP which acts on all four Rab3 isoforms and is broadly distributed in different tissues (26). It is now known to have two subunits including a p130 catalytic subunit. Mutations in p130 cause Warburg Micro syndrome in humans and deletion in mice affects transmitter release and neuroplasticity (59). There is no known specific Rab3 GDI, but rather a common family of Rab GDI isoforms which bind prenylated Rabs in the cytoplasm and participates in insertion and removal from membranes (75). Likewise, Rab Escort Protein plays a role in the recycling of many or all Rab proteins (2). Rab3A activity is also specifically regulated by calcium-calmodulin complex (15).
Considerable attention has been paid to understanding how Rab3A regulates secretion from neurons and neuroendocrine cells. Gene deletion of Rab3A had only subtle electrophysiological effects on transmitter release and long term potentiation (7, 28). Because of the presence of multiple forms of Rab3 in neuronal cells, the group of T. Sudhof prepared a mouse model in which all four isoforms of Rab3 were deleted. Although these mice died shortly after birth from respiratory failure (61), their cultured hippocampal neurons showed normal synaptic structure, but reduced transmitter release. This defect could be rescued by a single allele of Rab3A. In a study of chromaffin cells from the same compound knockout mice, the number of large dense core granules was reduced and the number of morphologically docked granules was normal, but the releasable pool was reduced indicating an altered function in priming (62). In a study of C. elegans which has a single Rab3 species, deletion reduced the number of synaptic vesicles at the neuromuscular junction and the amount of transmitter released (47). Another role of Rab3 mediated by its effector Rim is to localize secretion to sites of calcium channels in the presynaptic membrane (27). Overall these studies in neuronal cells indicate Rab3 is involved in vesicle formation and transmitter release. Rab3 has also been reported as a presynaptic target for ethanol sensitivity (4).
Little is known as to how Rab3 exerts its actions. Earlier work focused on the effects of Rab3 effector domain peptides, but these effects were probably the result of peptide insertion into membranes and may not reflect the activity of the intact Rab3 molecule. Current work focuses on the identification of specific Rab effector molecules and how they affect secretion at the molecular level. These possible effectors include syaptotagmin like proteins (Slp1-5), Slp proteins lacking C2 domains (Slac2), rabphillin, Rim, and Noc2 (25). These proteins contain a Rab-binding domain (RBD) which is sometimes referred to as a Slp homology domain. These proteins interact with Rab3 and Rab 27 isoforms and participate in the docking of secretory granules to the plasma membrane.
2. Rab3 and the Exocrine Pancreas
Small G proteins are known to be key regulators of pancreatic digestive enzyme secretion and Rab3 was one of the first to be studied (74). The primary form of Rab3 present in pancreatic acinar cells and on pancreatic ZG is Rab3D based on RT-PCR, Western blotting and mass spectrometry (11, 48, 69). Rab3D is also present in other exocrine glands with large secretory granules including parotid and other salivary glands, lacrimal acinar and gastric chief cells (12, 22,46,48,54,65). Immunohistochemistry localizes Rab3d to the ZG region of acinar cells with no obvious localization to ducts. High resolution confocal microscopy, immunogold electron microscopy, and mass spectrometry localized Rab3D to the outer surface of the ZG membrane where it is attached by isoprenyl groups (10,48,69). Over 90 % of Rab3D in mouse acini is particulate and most of this partitions into Triton X-114, indicating a hydrophobic component, in this case isoprenylation (9,69). Both immunohistochemistry of intact pancreas or acini and staining of granules after isolation indicates that essentially all ZG bear Rab3D as shown for rat and mouse pancreas in Figs 1 and 2. Following stimulation of secretion in rat pancreatic lobules, Rab3 (isoform unknown) was shown to redistribute from ZG to Golgi by EM immunohistochemistry (33). In contrast to rat and mouse pancreatic acinar cells, the AR42J cell line expresses all four forms of Rab3 and these show different density distributions (36,53). Subsequently, Rab3D in AR42J cells was shown to localize to ZG when dexamethasone was used to induce an acinar phenotype (39). Islet beta cells and beta cell derived cell lines also express all four forms of Rab3 (31,56).
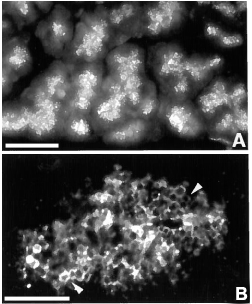
Figure 1. Immunoflourescence localization of Rab 3D in rat pancreas. A. Low power view (bar = 40 µm). B. High power shows outline of individual zymogen granules as indicated by arrowheads (bar = 10 µm) Reproduced from Reference (48).
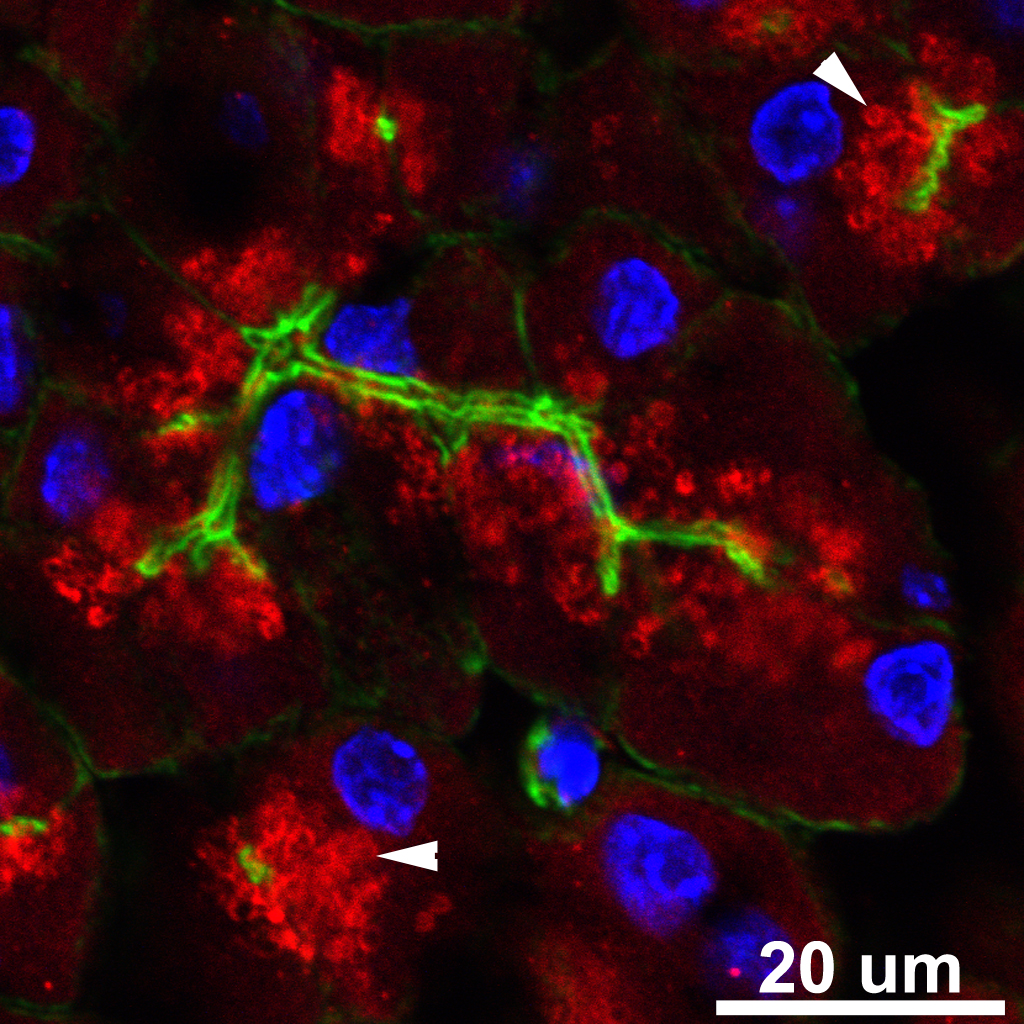
Figure 2. Mouse Pancreas triple labeled with anti Rab3D (Red), Phalloidin to stain filamentous actin (Green), and Dapi to stain nuclei (Blue). Arrowheads indicate individual ZG coated with Rab3D.
Developmental changes in acinar cell Rab3D have also been studied. Rab3D was first detectable in the embryonic rat pancreas at day 18, on day 20-21 was primarily cytosolic, with redistribution to a membrane fraction after birth (68). For reference, ZG appear between embryonic day 16-17 and regulated secretion becomes prominent shortly after birth. Rab3D also undergoes developmentally regulated carboxymethylation that decreases at the time when secretion begins (67). The same study showed that dexamethasone induced maturation of AR42J cells was accompanied by an increase in the unmethylated Rab3D protein.
As discussed in Section 1, most studies point to a role for Rab3 in regulating secretion and possibly exocytosis. The secretory granule localization of Rab3D also suggests a role in the secretory process. Early work showed putative effector domain peptides from Rab3A stimulated secretion from permeabilized acini and that this action synergized with GTPγS suggesting an effect on a terminal step in secretion (51). A similar effect was reported when ZGs were mixed with plasma membrane in vitro (20). Subsequent work however has focused on the intact structure and configuration of the G protein. A transgenic mouse study reported enhanced regulated amylase secretion from pancreatic acini of mice overexpressing epitope tagged Rab3D which was targeted to the zymogen granule (49). Because small G proteins can be locked into distinct guanine nucleotide bound configurations, X. Chen et al carried out studies using adenoviral vectors to overexpress mutant Rab3D forms in mouse acini (8). Overexpression of a dominant negative mutants Rab3D T36N or Rab3D N135I inhibited amylase secretion without affecting intracellular Ca2+ signaling while the constitutively active mutant Rab3D Q81L had no effect. Furthermore, the dominant negative mutants did not localize to the ZG, but rather in the basolateral region and upon cell fractionation to the cytosol. This combined with the fact that essentially 100% of Rab3D in acini under basal conditions is already in the GTP bound state, and that the dominant negative mutants reduced this to 25-35% as determined by a GST-Rim pull down assay, suggested that the mutants blocked GDP/GTP exchange (9). Thus the dominant negative Rab3D species could have been binding to a GEF acting on the related Rab protein, Rab 27B, raising questions of specificity that could better be answered by siRNA knockdown. However, such techniques are difficult on primary cells. In contrast to acini, only about 20% of Rab3A in islet beta cell is in the GTP bound active configuration, but can be increased by overexpressing Rab3 GEP (15).
A Rab3D knockout mouse has also been described (57). These mice did not have an obvious abnormal secretory function and both pancreas and parotid glands responded appropriately to secretagogues. However, the size of the secretory granules were increased and granule volume was calculated to be doubled. The authors concluded that rather than regulating secretion, Rab3D regulated granule formation. Mast cell granule exocytosis was also unaffected. Other evidence supporting a role for Rab3D in Golgi function and granule biogenesis is the finding of Rab3d on cis Golgi of goblet cells and Brunners gland acinar cells of the intestine (71). Another possible explanation of the findings in the Rab3D knockout mouse is that another Rab, such as Rab27B, was redundant with Rab3D and adapted to replace it. It will be interesting to see the result of a double Rab3D/Rab27B knockout.
Several putative effectors have been identified for mammalian pancreas Rab3 isoforms (25). Possible effectors for Rab3D include Noc2 (No C2 domain) and Slp1; Rabphillin and Slp-4/granulophillin are potential effectors for Rab3A in islet beta cells, but have not been reported to be present in acinar cells. GST-Noc2 interacts with GTP-liganded Rab3 and Noc2 knockout mice show acinar cells filled with zymogen granules, but with reduced amylase secretion; the mice also showed reduced insulin secretion (43). Antibody to Noc2 blocks secretion in permeabilized parotid acinar cells (32). However Noc2 may be mediating the effect of Rab27 rather than Rab3. Slp 1 can interact with Rab3D and also with Myosin 5 isoforms and may thereby regulate motility of granules; however, other Rabs also can play this role (18). Valentjin, Valentjin et al (70) suggested that Rab3D is involved in actin polymerization around exocytosing granules, but whether this is related to myosin is unknown. In islet beta cells, Rim2 interacting with Rab3A is required for granule docking and priming (77) while Slp-4 also known as granulophillin binds Rab3A and Munc-18 a SNARE protein regulator (14). Future work is necessary to establish the targets of Rab3D in pancreatic acinar cells.
3. Tools for the Study of Rab3
a. Antibodies
A number of antibodies are available including ones that react with all Rab3 isoforms and ones specific for individual species. To study Rab3D in pancreatic acini for Western blotting and immunofluorescence in frozen sections we have use a rabbit polyclona antisera prepared by Mark McNiven (Mayo Medical School) against a mouse peptide sequence from the c terminus of Rab3D (48). A similarly prepared antibody is available from Fitzgerald Industries (2OR-1343). Other antipeptide antibodies are available against the amino terminal sequence. A rabbit antibody against recombinant Rab3D has been prepared and used by Robert Raffaniello (39).
b. Plasmid and Viral Vectors
Plasmids coding for human Rab3A, 3B, and 3D with a 3xHA amino terminal epitope tag in pcDNA3.1 are available from Missouri Science & Technology cDNA Resource center (www.cdna.org). Adenoviral vectors coding for WT Rab3D and Q81L, N135I, and T36N mutants have been prepared by us and described (8).
c. Mouse Models
Knockout mice with Rab3D deleted on a C57BL6/J background have been prepared and described (57).
4. References
- Adachi R, Nigam R, Tuvim MJ, DeMayo F, and Dickey BF. Genomic organization, chromosomal localization, and expression of the murine RAB3D gene. Biochem and Biophys Res Comms 273: 877-883, 2000. PMID: 10891340
- Alexandrov K, Horiuchi H, Steele-Mortimer O, Seabra MC, Zerial M. Rab escort protein-1 is a multifunctional protein that accompanies newly prenylated rab proteins to their target membranes. EMBO 13: 5262-5273, 1994. PMID: 7957092
- Baldini G, Baldini G, Wang G, Weber M, Zweyer M, Bareggi R, Witkin JW, and Martelli AM. Expression of Rab3D N135I Inhibits Regulated Secretion of ACTH in AtT-20 Cells. J Cell Biol 140: 305-313, 1998. PMID: 9442106
- Barclay JW, Graham ME, Edwards MR, Johnson JR, Morgan A, and Burgoyne RD. Presynaptic targets for acute ethanol sensitivity. Biochem Soc Trans 38: 172-176, 2010. PMID: 20074054
- Baumert M, Fischer von Mollard G, Jahn R, and Sudhöf TC. Structure of the murine rab3A gene: correlation of genomic organization with antibody epitopes. Biochem J 293: 157-163, 1993. PMID: 7687127
- Brondyk WH, McKiernan CJ, Burstein ES, and Macara IG. Mutants of Rab3A Analogous to Oncogenic Ras Mutants. J Biol Chem 268: 9410-9415, 1993. PMID: 8387493
- Castillo PE, Janz R, Südhof TC, Tzounopoulos T, Malenka RC, and Nicoll RA. Rab3A is essential for mossy fibre long-term potentiation in the hippocampus. Nature 388: 590-593. PMID: 9252190
- Chen X, Edwards JAS, Logsdon CD, Ernst SA, and Williams JA. Dominant negative Rab3D inhibits amylase release from mouse pancreatic acini. J Biol Chem 277: 18002-18009, 2002. PMID: 11875077
- Chen X, Ernst SA, and Williams JA. Dominant negative Rab3D mutants reduce GTP-bound endogenous Rab3D in pancreatic acini. J Biol Chem 278: 50053-50060, 2003. PMID: 14522985
- Chen X, Ulintz PJ, Simon ES, Williams JA, and Andrews PC. Global Topology Analysis of Pancreatic Zymogen Granule Membrane Proteins. Mol & Cell Prot 7.12: 2323-2336, 2008. PMID: 18682380
- Chen X, Walker AK, Strahler JR, Simon ES, Tomanicek-Volk SL, Nelson BB, Hurley MC, Ernst SA, Williams JA, and Andrews PC. Organellar Proteomics: Analysis of Pancreatic Zymogen Granule Membranes. Mol Cel Prot 5.2: 306-312, 2006. PMID: 16278343
- Chiang L, Ngo J, Schechter JE, Karvar S, Tolmachova T, Seabra MC, Hume AN, Hamm-Alvarez SF. Rab27b regulates exocytosis of secretory vesicles in acinar epithelial cells from the lacrimal gland. Am J Physiol Cell Physiol 302: C507-C521, 2010. PMID: 21525430
- Conner S, and Wessel GM. Rab3 mediates cortical granule exocytosis in the sea urchin egg. Dev Biol 203: 334-344, 1998. PMID: 9808784
- Coppola T, Frantz C, Perret-Menoud V, Gattesco S, Hirling H, and Regazzi R. Pancreatic β-cell protein granuphilin binds Rab3 and Munc-18 and controls exocytosis. Mol Biol Cell 13: 1906-1915, 2002. PMID: 12058058
- Coppola T, Perret-Menoud V, Gattesco S, Magnin S, Pombo I, Blank U, and Regazzi R. The death domain of Rab3 guanine nucleotide exchange protein in GDP/GTP exchange activity in living cells. Biochem J 362: 273-279, 2002. PMID: 11853534
- Coppola T, Perret-Menoud V, Lüthi S, Farnsworth CC, Glosmet JA, and Regazzi R. Disruption of Rab3-calmodulin interaction, but not other effector interactions, prevents Rab3 inhibition of exocytosis. EMBO 18: 5885-5891, 1999. PMID: 10545100
- Demo SD, Msuda E, Rossi AB, Throndset BT, Gerard AI, Chan EH, Armstrong RJ, Fox BP, Lorens JB, Payan DG, Scheller RH, and Fisher JM. Quantitative measurement of mast cell degranulation using a novel flow cytometric annexin-V binding assay. Cytometry 36: 340-348, 1999. PMID: 10404150
- Deneka M, Neeft M, and van der Sluijs P. Regulation of membrane transport by rab GTPases. Critical Reviews in Biochemistry and Molecular Biology 38: 121-142, 2003. PMID: 12749696
- Dumas JJ, Zhu Z, Connolly JL, and Lambright DG. Structural basis of activation and GTP hydrolysis in Rab proteins. Structure 7: 413-423, 1999. PMID: 10196122
- Edwardson JM, MacLean CM, and Law GJ. Synthetic peptides of the rab3 effector domain stimulate a membrane fusion event involved in regulated exocytosis. FEBS Letters 320: 52-56, 1993. PMID: 8385025
- Evans E, Zhang W, Jerdeva G, Chen C-Y, Chen X, Hamm-Alvarez SF, and Okamoto CT. Direct interaction between Rab3D and the polymeric immunoglobulin receptor and trafficking through regulated secretory vesicles in lacrimal gland acinar cells. Am J Physiol Cell Physiol 294: C662-C674, 2008. PMID: 18171724
- Field RB, Kruse DH, and Redman RS. Immunohistochemical localization and mRNA detection of Rab3D and/or Rab3B in rat von Ebner’s glands, parotid gland, pancreas, and liver. Histochem J 33: 71-77, 2001. PMID: 11432642
- Figueiredo AC, Wasmeier C, Tarafder AK, Ramalho JS, Baron RA, and Seabra MC. Rab3GEP is the non-redundant guanine nucleotide exchange factor for Rab27a in melanocytes. J Biol Chem 283: 23209-23216, 2008. PMID: 18559336
- Fisher von Mollard G, Mignery GA, Baumert M, Perin MS, Hanson TJ, Burger PM, Jahn R, and Südhof TC. Rab3 is a small GTP-binding protein exclusively localized to synaptic vesicles. Proc Natl Acad Sci USA 87: 1988-1992, 1990. PMID: 2155429
- Fukuda M. Regulation of secretory vesicle traffic by Rab small GTPases. Cell Mol Life Sci 65: 2801-2813, 2008. PMID: 18726178
- Fukui K, Sasaki T, Imazumi K, Matsuura Y, Nakanishi H, and Takai Y. Isolation and characterization of a GTPase activating protein specific for the Rab3 subfamily of small G proteins. J Biol Chem 272: 4655-5658, 1997. PMID: 9030515
- Gandini MA, Felix R. Functional interactions between voltage-gated Ca(2+) channels and Rab3-interacting molecules (RIMs): New insights into stimulus-secretion coupling. Biochim Biophys Acta 1818: 551-558, 2012. PMID: 22198390
- Geppert M, Goda Y, Stevens CF, and Südhof TC. The small GTP-binding protein Rab3A regulates a late step in synaptic vesicle fusion. Nature 387: 810-814, 1997. PMID: 9194562
- Gevrey J, Laurent S, Saurin J, Némoz-Gaillard E, Regazzi R, Chevrier A, Chayvialle J, and Abello J. Rab3 controls exocytosis in cholecystokinin-secreting cells. FEBS Letters 503: 19-24, 2001. PMID: 11513847
- Holz RW, Brondyk WH, Senter RA, Kuizon L, and Macara IG. Evidence for the Involvement of Rab3A in Ca2+-dependent exocytosis from adrenal chromaffin cells. J Biol Chem 269: 10229-10234, 1994. PMID: 8144603
- Iezzi, M, Escher G, Meda P, Charollais A, Baldini G, Darchen F, Wollheim CB, and Regazzi R. Subcellular distribution and function of Rab3A, B, C, and D isoforms in insulin-excreting cells. Mol Endo 13: 202-212, 1999. PMID: 9973251
- Imai A, Yoshie S, Nashida T, Shimomura H, and Fukuda M. Functional involvement of Noc2, a Rab27 effector, in rat parotid acinar cells. Arch of Biochem Biophys 455: 127-135, 2006. PMID: 17067543
- Jena BP, Gumkowski FD, Konieczko EM, Fischer von Mollard G, Jahn R, and Jamieson JD. Redistribution of Rab3-like GTP-binding protein from secretory granules to the golgi complex in pancreatic acinar cells during regulated exocytosis. J Cell Biol 124: 43-53, 1994. PMID: 8294505
- Johannes L, Lledo P, Roa M, Vincent J, Henry J, and Darchen F. The GTPase Rab3a negatively controls calcium-dependent exocytosis in neuroendocrine cells. EMBO 13: 2029-2037, 1994. PMID: 8187757
- Johnston PA, Archer BT 3rd, Robinson K, Mignery GA, Jahn R, and Südhof TC. Rab3A attachment to the synaptic vesicle membrane mediated by a conserved polyisoprenylated carboxy-terminal sequence. Neuron 7: 101-109, 1991. PMID: 1648935
- Klengel R, Piiper A, Pittelkow S, and Zeuzem S. Differential expression of Rab3 isoforms during differentiation of pancreatic acinar cell line AR42J. Biochem and Biophys Res Comm 236: 719-722, 1997. PMID: 9245721
- Knop M, Aareskjold E, Bode G, and Gerke V. Rab3D and annexin A2 play a role in regulated secretion of vWF, but not tPA, from endothelial cells. EMBO 23: 2982-2992, 2004. PMID: 15257287
- Larkin JM, Woo B, Balan V, Marks DL, Oswald BJ, LaRusso NF, and McNiven MA. Rab3D, a small GTP-binding protein implicated in regulated secretion, is associated with the transcytotic pathway in rat hepatocytes. Hepatology 32: 348-356, 2000. PMID: 10915742
- Limi S, Ojakian G, and Raffaniello R. Rab3D regulates amylase levels, not agonist-induced amylase release, in AR42J cells. Cellular & Molecular Biology Letters 17: 258-273, 2012. PMID: 22367855
- Lledo P, Vernier P, Vincent J, Mason WT, and Zorec R. Inhibition of Rab3B expression attenuates CA(2+)-dependent exocytosis in rat anterior pituitary cells. Nature 364: 540-544, 1993. PMID: 8393147
- Luo HR, Saiardi A, Nagata E, Ye K, Yu H, Jung TS, Luo X, Jain S, Sawa A, and Snyder SH. GRAB: a physiologic guanine nucleotide exchange factor for Rab3A, which interacts with inositol hexakisphosphate kinase. Neuron 31: 439-451, 2001. PMID: 11516400
- Martelli AM, Baldini G, Tabellini G, Koticha D, Bareggi R, and Baldini G. Rab3A and Rab3D control the total granule number and the fraction of granules docked at the plasma membrane in PC12 cells. Traffic 1: 976-986, 200. PMID: 11208087
- Matsumoto M, Miki T, Shibasaki T, Kawaguchi M, Shinozaki H, Nio J, Saraya A, Koseki H, Miyazaki M, Iwanaga T, and Seino S. Noc2 is essential in normal regulation of exocytosis in endocrine and exocrine cells. Proc Natl Acad Sci USA 101: 8313-8318, 2004. PMID: 15159548
- Michaut M, Tomes CN, De Blas D, Yunes R, and Mayorga LS. Calcium-triggered acrosomal exocytosis in human spermatozoa requires the coordinated activation of Rab3A and N-ethylmaleimide-sensitive factor. Proc of the Natl Acad Sci USA 97: 9996-10001, 2000. PMID: 10954749
- Miller AL, Pavlos NJ, Xu J, and Zheng MH. Rab3D: a regulator of exocytosis in non-neuronal cells. Histol Histopathol 17: 929-936, 2002. PMID: 12168804
- Ngyen D, Jones A, Ojakian GK, and Raffaniello RD. Rab3D Redistribution and Function in Rat Parotid Acini. J Cell Physiol 197:400-408, 2003. PMID: 14566969
- Nonet ML, Staunton JE, Kilgard MP, Fergestad T, Hartwieg E, Horvitz R, Jorgensen EM, and Meyer BJ. Caenorhabditis elegens rab-3 mutant synapses exhibit impaired function and are partially depleted of vesicles. Neuroscience 17: 8061-8073, 1997. PMID: 9334382
- Ohnishi H, Ernst SA, Wys N, McNiven M, and Williams JA. Rab3D localizes to zymogen granules in rat pancreatic acini and other exocrine glands. Am J Physiol Gastro Liver Physiol 271: G531-G538, 1996. PMID: 8843780
- Ohnishi H, Samuelson LC, Yule DI, Ernst SA, and Williams JA. Overexpression of Rab3D enhances regulated amylase secretion from pancreatic acini of transgenic mice. J Clin Invest 100: 3044-3052, 1997. PMID: 9399951
- Ostermeier C, and Brunger AT. Structural basis of Rab effector specificity: crystal structure of the small G protein Rab3A complexed with the effector domain of Rabphilin-3A. Cell 96: 363-374, 1999. PMID: 10025402
- Padfield PJ, Balch WE, Jamieson JD. A synthetic peptide of the rab3a effector domain stimulates amylase release from permeabilized pancreatic acini. Proc Natl Acad Sci USA 89: 1656-1660, 1992. PMID: 1371881
- Pavlos NJ, Xu J, Riedl D, Yeoh JSG, Teitelbaum SL, Papadimitriou JM, Jahn R, Ross FP, Zheng MH. Rab3D regulates a novel vesicular trafficking pathway that is required for osteoclastic bone resorption. Mol Cell Biol. 25: 5253-5269, 2005.
- Piiper A, Leser J, Lutz MP, Beil M, and Zeuzem S. Subcellular distribution and function of Rab3A-D in pancreatic acinar AR42J cells. Biochem and Biophys Res Comm 287: 746-751, 2001. PMID: 11563859
- Raffaniello RD, Lin J, Wang F, and Raufman J. Expression of Rab3D in dispersed chief cells from guinea pig stomach. Biochim Biophys Acta 1311: 111-116, 1996. PMID: 8630328
- Roa M, Paumet F, Le Mao J, David B, Blank U. Involvement of the ras-like GTPase rab3d in RBL-2H3 mast cell exocytosis following stimulation via high affinity IgE receptors (FcεRI). J Immunol 159: 2815-2823, 1997.
- Regazzi R, Ravazzola M, Iezzi M, Lang J, Zahraoui A, Anderggen E, Morel P, Takai Y, and Wolheim C. Expression, localization and functional role of small GTPases of the Rab3 family in insulin-secreting cells. Cell Science 109: 2265-2273, 1996. PMID: 8886977
- Riedel D, Antonin W, Fernandez-Chacon, Alvarez de Toledo G, Jo T, Geppert M, Valentijn JA, Valentijn K, Jamieson JD, Südhof TC, and Reinhard J. Rab3D is not required for exocrine exocytosis but for maintenance of normally sized secretory granules. Molecular and Cellular Biology 22: 6487-6497, 2002. PMID: 12192047
- Rupnik M, Kreft M, Nothias F, Grilc S, Bobanovic LK, Johannes L, Kiauta T, Vernier P, Darchen F, and Zorec R. Distinct role of Rab3A and Rab3B in secretory activity of rat melantrophs. Am J Physiol Cell Physiol 292: C98-C105, 2007. PMID: 16822953
- Sakane A, Manabe S, Ishizaki H, Tanaka-Okamoto M, Kiyokage E, Toida K, Yoshida T, Miyoshi J, Kamiya H, Takai Y, and Sasaki T. Rab3 GTPase-activating protein regulates synaptic transmission and plasticity through the inactivation of Rab3. Proc Natl Acad Sci USA 103: 10029-10034, 2006. PMID: 16782817
- Schlüter OM, Khvotchev M, Jahn R, and Südhof TC. Localization versus function of Rab3 Proteins. J Biol Chem 277: 40919-40929, 2002. PMID: 12167638
- Schlüter OM, Schmitz F, Rosenmund C, and Südhof TC. A complete genetic analysis of neuronal Rab3 function. The Journal of Neuroscience 24: 6629-6637, 2004. PMID: 15269275
- Schonn J, van Weering JR, Mohrmann R, Schülter OM, Südhof TC, de Wit H, Verhage M, and Sørensen JB. Rab3 proteins involved in vesicle biogenesis and priming in embryonic mouse chromaffin cells. Traffic 11: 1415-1428, 2010. PMID: 20716109
- Stenmark H. Rab GTPases as coordinators of vesicle traffic. Nature Reviews Molecular Cell Biology 10: 513-525, 2009. PMID: 19603039
- Tanaka M, Miyoshi J, Ishizaki H, Togawa A, Ohnishi K, Endo K, Matsubara K, Mizoguchi A, Nagano T, Sato M, Sasaki T, and Takai Y. Role of Rab3 GDP/GTP exchange protein in synaptic vesicle trafficking at the mouse neuromuscular junction. Mol Biol Cell 12: 1421-1430, 2001. PMID: 11359932
- Tang LH, Gumkowski FD, Sengupta D, Modlin IM, and Jamieson JD. Rab3D protein is a specific marker for zymogen granules in gastric chief cells of rats and rabbits. Gastroenterology 110: 809-820, 1996. PMID: 8608891
- Tuvim MJ, Adachi R, Chocano JF, Moore RH, Lampert RM, Zera E, Romero E, Knoll BJ, and Dickey BF. Rab3D, a small GTPase is localized on mast cell secretory granules and translocates to the plasma membrane upon exocytosis. Am J Respir Cell Mol Biol 20: 79-89, 1999. PMID: 9870920
- Valentijn JA, and Jamieson JD. Carboxyl methylation of rab3D is developmentally regulated in the rat pancreas: correlation with exocrine function. Eur J of Cell Biol 76: 204-211, 1998. PMID: 9716267
- Valentijn JA, Gumkowski FD, and Jamieson JD. The expression pattern of rab3D in the developing rat exocrine pancreas coincides with the acquisition of regulated exocytosis. Eur J of Cell Biol 71: 129-136, 1996. PMID: 8905289
- Valentijn JA, Sengupta D, Gumkowski FD, Tang LH, Konieczko EM, and Jamieson JD. Rab3D localizes to secretory granules in rat pancreatic acinar cells. Eur J of Cell Biol 70: 33-41, 1996. PMID: 8738417
- Valentijn JA, Valentijn K, Pastore LM, and Jamieson JD. Actin coating of secretory granules during regulated exocytosis correlates with the release of rab3D. Proc Natl Acad Sci USA 97: 1091-1095, 2000. PMID: 10655489
- Valentijn JA, van Weeren L, Ultee A, and Koster AJ. Novel localization of Rab3D in rat intestinal goblet cells and Brunner’s gland acinar cells suggests a role in early Golgi trafficking. Am J Physiol Gastrointest Liver Phsyiol 293: G165-G177, 2007. PMID: 17395899
- Wada M, Nakanishi H, Satoh A, Hirano H, Obaishi H, Matsuura Y, and Takai Y. Isolation and characterization of a GDP/GTP exchange protein specific for the Rab3 subfamily small G proteins. J Biol Chem 272: 3875-3878, 1997. PMID: 9020086
- Weber E, Jilling T, and Kirk K. Distinct Functional Properties of Rab3A and Rab3B in PC12 Neuroendocrine Cells. J Biol Chem 271: 6963-6971, 1996. PMID: 8636125
- Williams JA, Chen X, and Sabbatini ME. Small G proteins as key regulators of pancreatic digestive enzyme secretion. Am J Physiol Endocrinol Metab 296: E405-E414, 2009. PMID: 19088252
- Wu S, Zeng K, Wilson IA, and Balch WE. Structural insights into the function of the Rab GDI superfamily. Trends Biochem Sci 21: 472-476, 1996. PMID: 9009830
- Yaekura K, Julyan R, Wicksteed BL, Hays LB, Alarcon C, Sommers S, Poitout Vm Baskin DG, Wang Y, Philipson LH, and Rhodes CJ. Insulin secretory deficiency and glucose intolerance in Rab3A null mice. J Biol Chem 278: 9715-9721, 2003. PMID: 12510060
- Yasuda T, Shibasaki T, Minami K, Takahashi H, Mizoguchi A, Uriu Y, Numata T, Mori Y, Miyazaki J, Miki T, and Seino S. Rim2alpha determines docking and priming states in insulin granule exocytosis. Cell Metab 12: 117-29, 2010. PMID: 20674857
- Zerial M, and McBride H. RAB proteins as membrane organizers. Nature Reviews Molecular Cell Biology 2: 107-119, 2001. PMID: 11252952
- Zografou S, Basagiannis D, Papafotika A, Shirakawa R, Horiuchi H, Auerbach D, Fukuda M, and Christoforidis S. A complete Rab screening reveals novel insights in Weibel-Palade body exocytosis. J Cell Sci 125: 4780-4790, 2012. PMID: 22899725