Entry Version:
Citation:
Pancreapedia: Exocrine Pancreas Knowledge Base, DOI: 10.3998/panc.2011.12
Attachment | Size |
---|---|
![]() | 467.64 KB |
Gene symbols: Sctr
1. General Structure and Functions
Secretin and its receptor hold special places in history, with secretin representing the first “hormone”, giving rise to the field of endocrinology when W. M. Bayliss and E. H. Starling recognized it as a blood-borne non-neural mediator of pancreatic exocrine secretion in 1902 (1), and with the secretin receptor cloned by T. Ishihara et al. in 1991 (2), representing the first family B G protein-coupled receptor and giving rise to this new and important receptor family.
The secretin receptor is a guanine nucleotide-binding protein (G protein)-coupled receptor (GPCR) that binds to and is activated by the peptide hormone, secretin. This receptor was the first member of the B family of GPCRs (3, 4), which also includes receptors for vasoactive intestinal polypeptide (VIP), pituitary adenylate cyclase-activating peptide (PACAP), glucagon, glucagon-like peptide (GLP), glucose-dependent insulinotropic polypeptide (GIP), calcitonin, calcitonin-like peptide, parathyroid hormone (PTH), corticotrophin-releasing factor (CRF), and growth hormone releasing hormone (GHRH) (3, 4). It is notable that these receptors lack the classical signature sequences found in most members of the much larger A family of GPCRs (5, 6). The most typical features of B family GPCRs includes a long amino-terminal tail region with six highly conserved cysteine residues that contribute to three structurally and functionally important intra-domain disulfide bonds, and seven hydrophobic transmembrane segments having residue conservation quite distinct from other family GPCRs (Fig. 1, left panel) (5-7).
In evaluation of these sequences, it has been proposed that the helical bundle of family B GPCRs is structurally distinct from that of family A GPCRs (5-7), even though it still likely includes a heptahelical bundle conformation and is known to couple with the same group of heterotrimeric G proteins. It is noteworthy that family B GPCRs typically couple with both Gs and Gq, in contrast to family A GPCRs that often couple with only a single dominant G protein. The extracellular amino-terminal tail domains of several members of this receptor family have recently been structurally characterized, either by NMR or crystallography, to exhibit a highly conserved core that includes two anti-parallel β-sheets, three disulfide bonds, and variable amino-terminal α-helix and multiple loop regions (8-17). The structure also provides a conserved hydrophobic peptide-binding cleft above the stable core and between the helix and loop regions.
Like many GPCRs, the secretin receptor is glycosylated on ectodomains, predominantly within the amino-terminal tail, and also on the second extracellular loop (18). These all represent sites of N-linked glycosylation of asparagine residues. Site-directed mutagenesis has demonstrated potential functional importance of glycosylation on Asn72 and Asn291 of the human secretin receptor (Fig 1 shows Asn78 and Asn263 of the rat sequence.), representing the first of three glycosylation sites within the amino-terminal tail and the glycosylation site within the second extracellular loop, although selective, single mutation of the other sites had no demonstrable functional impact (18). Generally, the glycosylation sites of membrane receptors are important for proper folding during biosynthesis, ensuring normal trafficking, and for protecting the receptor from proteolytic degradation.
Desensitization of GPCRs often can be induced both by biochemical modifications such as phosphorylation and by cellular mechanisms such as internalization. The secretin receptor utilizes both of these. Like many receptors that activate intracellular kinases, phosphorylation of the secretin receptor is utilized as a biochemical mechanism for feedback regulation involved in desensitization. Direct evidence for agonist-stimulated phosphorylation of the secretin receptor was first demonstrated by Ozcelebi et al. (19). That work established the phosphorylation of threonine and serine residues within the receptor carboxyl-terminal tail region. The kinases involved in phosphorylation of the secretin receptor were found to represent predominantly G protein-coupled receptor kinases, with minor action by both protein kinase A and protein kinase C (20). Secretin receptor phosphorylation has been shown to disrupt G protein coupling (21), but not to be necessary for agonist-induced receptor internalization (21). The latter certainly occurs, and represents a major mechanism for desensitization of this receptor.
In recent years, it has been recognized that certain GPCRs can associate with themselves and with structurally related GPCRs to form oligomeric or dimeric complexes (22). While most of these studies have been directed toward family C or family A GPCRs, recent work has shown that it is relevant to family B GPCRs as well (22). The evidence is best for family C GPCRs, where dimeric structures typically have profound effects on receptor function and specificity, and where crystal structures of covalently-bonded dimeric complexes of ectodomains have been reported (23). In family A GPCRs, there have been no consistent observations of which receptors might associate with themselves or other GPCRs, of functional effects of oligomerization, or of the impact of ligand binding on receptor oligomerization (23). The secretin receptor is among the best studied family B GPCR in regard to its oligomerization. It is constitutively present within homo-dimeric complexes determined by the lipid-exposed face of the fourth transmembrane segment (24-26). This structure is critically important for achieving the high affinity G protein-coupled state of this receptor, with non-dimerizing mutants coupling less efficiently, binding secretin with lower affinity, and having less potent effects of secretin to stimulate cAMP (24-26). Of note, only the dimeric state of this receptor exhibits negative cooperativity (24), another protective feature of some receptor systems. The structurally specific nature of secretin receptor homo-dimers appears to be consistent throughout this receptor family (27). This receptor is also able to form hetero-dimers with other family B GPCRs (28).
There is also a single report to demonstrate the ability of the single transmembrane Receptor Activity-Modifying Protein-3 (RAMP-3) to associate with the secretin receptor during its biosynthesis (29). Of note, since the secretin receptor is able to traffick to the cell surface independent of its association with RAMPs, it is difficult to identify a specific effect of this bimolecular association, unlike those members of the B family of GPCRs that cannot get to the cell surface without RAMPs, such as the calcitonin receptor-like receptor (30). Indeed, no specific functional effect has yet been demonstrated for RAMP-3 association with the secretin receptor (29).
Like secretin, all of the natural ligands of family B GPCRs, represent moderately long peptide hormones having diffuse pharmacophoric domains (3, 4). These all have the propensity to form helical structures, particularly in their carboxyl-terminal ends, a region of these peptides now known to bind to the hydrophobic cleft within the amino terminus of their receptors. This binding directs the amino-terminal region of these ligands toward the core helical bundle domain of their receptors. This portion of secretin and other family B GPCR ligands is responsible for its biological activity, with amino-terminally truncated forms of these ligands acting as antagonists (31-33). The right panel of Figure 1 illustrates the current working model of secretin occupation of its binding site within the secretin receptor.
The classical effects of secretin are the stimulation of the secretion of bicarbonate, water, and electrolytes from the pancreatic ductular system, as well as the biliary ducts (34, 35). These effects are largely stimulated by secretin released from S cells in the proximal intestine in response to intraluminal acid and fatty acids, thus playing a hormonal regulatory role and a classical feedback loop (35). Subsequently, it has become clear that this receptor is also expressed in many other tissues, and that it has pleiotrophic roles (36). Of particular interest, some of these roles are mediated by neuronal secretin, thus acting as a neurotransmitter (37). Many of the secretin receptors in brain and kidney play important roles related to fluid homeostasis (38). Prominent expression in brain has been described in cerebellum, with intermediate levels of expression in cortex, thalamus, striatum, hippocampus and hypothalamus, and low levels of expression in midbrain, medulla, and pons (36, 39). Secretin has been described to stimulate drinking behavior via central nervous system actions that include release of vasopressin (38, 40). Secretin receptors are expressed on essentially all cells within the kidney, with this hormone described to stimulate renal blood flow and glomerular filtration rate, as well as tubular water reabsorption, and to have effects on translocation of aquaporin (38, 40). Secretin has been described to have both diuretic and anti-diuretic effects, dependent on dose and route of administration (41). Secretin receptors are also expressed on gastrointestinal smooth muscle, neurons, and certain mucosal cells, heart, and lung (39).
Like most family B GPCRs, the secretin receptor is known to couple to both Gs and Gq, with the cAMP response to stimulation most prominent and sensitive, while the intracellular calcium responses are only observed with high concentrations of hormone (42). The determinants for G protein coupling have been best studied for family A GPCRs, where distinct motifs have been present in the juxtamembranous regions of the second and third intracellular loops and carboxyl-terminal tail (43, 44). Some work has been reported for family B GPCRs (45-47), but the same motifs in the same positions appear to be absent. It is interesting that the determinants for coupling with these two G proteins are apparently both shared and distinct, with mutations able to disrupt both Gs and Gq coupling, as well as more selective disruption of some receptor mutations (47). Like many GPCRs, full signaling responses are recorded with less than 20 percent of receptors occupied (48, 49). There are typically spare receptors present on physiologic targets of this hormone, but there have been no detrimental effects observed for stimulation with supramaximal concentrations of secretin. These signaling pathways have prominent effects on cell surface transporters involved in fluid and electrolyte transport (38). Stimulation of this receptor has also been observed to affect translocation and activation of various aquaporins in different tissues, consistent with its important roles in fluid homeostasis (38, 40).
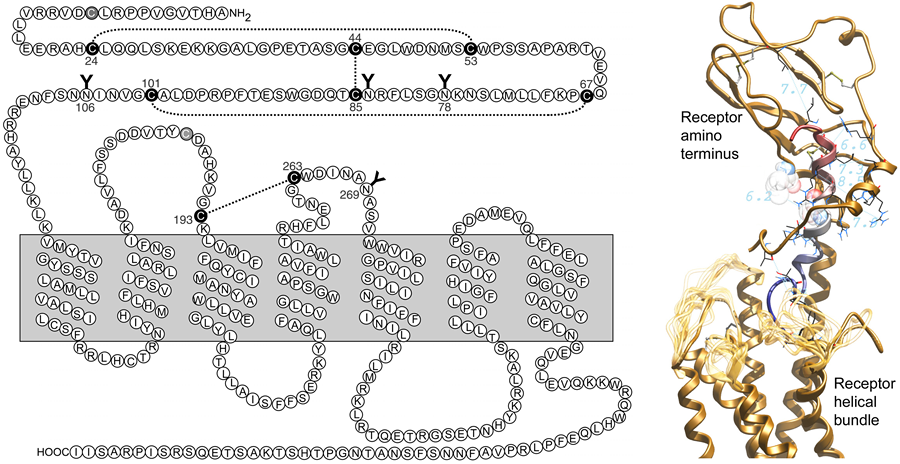
Figure 1. Shown are a two-dimensional schematic diagram of the secretin receptor and a three-dimensional molecular model of the secretin occupied receptor. In the left panel, the primary amino acid sequence of the rat secretin receptor is illustrated along with the proposed topology with seven transmembrane segments, a glycosylated and disulfide-bonded extracellular amino-terminal tail, extracellular and intracellular loops, and an intracellular carboxyl-terminal tail. There is also a disulfide bond linking cysteines within the first and second extracellular loops, and another site of glycosylation in the second extrcellular loop. In the right panel, the secretin peptide ligand is shown colored blue to red from the amino terminus, directed into the intramembranous helical bundle region, to the carboxyl terminus, occupying the peptide-binding cleft within the receptor amino terminus. The receptor is illustrated in gold, with the amino-terminal domain above the transmembrane helical bundle. The loop regions reflect the possible variability in that part of the current model in the absence of additional constraints. Right panel derived from a figure published in Journal of Biological Chemistry 285:9919-9931, 2010 (75), with permission of the American Society for Biochemistry and Molecular Biology.
While secretin receptors have been reported to be present on various neoplasms, including ductular carcinomas of the pancreas and selected islet cell carcinomas (50), it is not clear that the integrity of the receptor or the signaling responses to secretin are normal in those cells. In fact, the splicing of the secretin receptor mRNA has been reported to be abnormal in some of these tumors (51-55). A series of secretin receptor spliceoforms have been described (50). This includes the skipping of exon 4, exons 3 and 4, exons 2 and 3, and exon 9. The exon 3 skipping first observed in a gastrin-secreting islet cell tumor, and later in pancreatic ductal carcinomas and cholangiocarcinomas, has been reported to have no intrinsic activity and even to exhibit dominant negative activity to inhibit the function of wild type receptor (54). This has the possible effect to reduce a growth inhibitor, thus stimulating neoplastic growth (50).
2. Specific Functions in the Pancreas
The pancreatic effects of secretin are, indeed, the most extensively studied and best described (56). This hormone was first recognized based on its ability to stimulate pancreatic exocrine secretion. Secretin receptors are present on pancreatic ductular cells, acinar cells, and even some cells within the islets (51, 57). They are also present on a number of pre-neoplastic and neoplastic conditions, including panin lesions (pancreatic intraductal neoplasia), ductal adenocarcinomas, intraductal papillary mucinous tumor syndrome, serous cystic tumors, and some islet cell tumors (51).
The major pancreatic function of secretin is to stimulate the secretion of bicarbonate, water, and electrolytes (34, 35, 56, 58, 59). This is mediated by receptors present on the basolateral surface of ductular cells, where stimulation results in activation of cAMP and protein kinase A. This, in turn, activates cystic fibrosis transmembrane regulator to affect chloride flux, resulting in membrane depolarization. This increases the force of the electrogenic sodium-bicarbonate cotransporter that increases cytosolic bicarbonate and then secretes it at the apical membrane through the chloride/bicarbonate exchanger. As noted above, this effect is controlled by secretin secreted from intestinal S cells that are stimulated by intraluminal acidic chyme. The result of this alkaline secretion then neutralizes the acidic chyme, helping to establish an optimal milieu for the action of pancreatic and intestinal enzymes involved in the digestion of protein, lipid, and carbohydrate and their subsequent absorption. Of note, secretin also has a trophic effect on ductal cells, with biliary ductular proliferation observed upon stimulation with high concentrations of secretin; this is not seen with secretin administration to secretin receptor knockout mice (60). Whether a similar effect occurs in the pancreas is unknown.
At the acinar cell, secretin stimulation can result in a modest secretion of zymogen content, including amylase, in some species (61). It is noteworthy that this effect is weak in most species, with the rat acinar cell typical of this (48), and with the guinea pig acinar cell exhibiting a substantially greater response (49). It has also been described that secretin can potentiate the exocrine secretory effect of cholecystokinin (62). Secretin has also been described to modulate insulin and glucagon release from B and A cells within the islet (63-65).
3. Tools to Study the Secretin Receptor
a. Molecular constructs
Mouse, rat, and human wild type secretin receptor cDNA clones in pDONR vector can be purchased from GeneCopoeia (www.genecopoeia.com). Human secretin receptor cDNA clone in pcDNA3.1 vector is available from UMR cDNA Resource Center, Missouri University of Science and Technology (www.cdna.org). Our laboratory uses rat and human secretin receptor cDNA clones in pcDNA 3.0. Additionally, a human secretin receptor splice variant missing exon 3 in pcDNA3.0 (54) and another variant missing both exon 3 and 4 in pBluescript KS+ vector (55) have been prepared in our laboratory.
b. Antibodies
Polyclonal anti-secretin receptor antibodies raised against the full-length receptor or its peptide fragments are commercially available from a number of vendors, including Abbiotec (250854), Abcam (ab85565 and ab58654), Abnova Corp. (H00006344-B01 and PAB5567), Acris Antibodies GmbH (AP08768PU-N), Atlas Antibodies (HPA007269), GenWay Biotech, Inc. (18-783-77147-1 ml), Insight Genomics (RPA47872), LifeSapn BioSciences (LS-B2374-50), Novus Biologicals (H00006344-B01), Raybiotech, Inc. (129-10604), R&D Systems (AF6387), Santa Cruz Biotechnology, Inc. (sc-26633 and sc-26632), Sigma –Aldrich (S8448 and HPA007269). A monoclonal antibody clone is available from Abnova Corp. and Novus Biologicals (the same catalogue number for both: H00006344-M01). However, we have not tested any of these in our laboratory. We were able to insert a hemagglutinin (HA) tag in position 37 of the mature secretin receptor (66) and have used anti-HA antibody for immunostaining and Western blot studies. Dr. B. K. Chow used an antibody raised against a mouse secretin receptor fragment for immunofluorescence staining in his laboratory (40). Additionally, two monoclonal antibodies directed against a secretin receptor splice variant missing both exons 3 and 4 have been generated in our laboratory (55).
c. Transgenic mice
Secretin receptor knockout mice (SCTR-/-) have been developed in the laboratories of Dr. B. K. Chow (67) and Dr. I. Nishijima (68). These SCTR-/- mice are described to be overtly normal and to be normally fertile. However, upon focused evaluation, they have altered water reabsorption in the renal tubules due to reduced expression of urine concentration-regulating proteins, aquaporin 2 and 4, in the kidney (67). They also have impaired synaptic plasticity in the hippocampus and slightly fewer dendritic spines in the CA1 hippocampal pyramidal cells, as well as exhibiting abnormal cognitive and social behaviors (68).
d. Activity
Binding affinity of the secretin receptor is determined by radioligand competition binding assay using a secretin radioligand and membranes prepared from cells expressing the secretin receptor (69). Currently, there is not a commercial source of radiolabeled secretin for such assays. Oxidative labeling of natural secretin has been described, using strong oxidation for an extended time to incorporate the radioiodine into the histidine in position 1 (70). However, this residue is critical for receptor binding and this radiolabel is not useful for receptor binding. Similarly, Bolton-Hunter reagent has been used to label the amino terminus of secretin (71) with the same negative effect on receptor binding. A simple oxidative radioiodination method is applicable to an analogue of secretin incorporating a tyrosine into position ten (72). This technique is well described in that paper (72).
Extensive photoaffinity labeling of the secretin receptor has also been performed (73-78). Sites of covalent attachment throughout the diffuse pharmacophore have been utilized, with many of these identifying residues within the receptor amino-terminal peptide-binding cleft (73-75, 78), and with a few sites at the amino terminus of the secretin ligand labeling the receptor core helical bundle domain (76, 77). Techniques for photoaffinity labeling are reviewed in The Pancreapedia Research Tools page by Dong and Miller.
Ligand binding induces conformational changes in the receptor, leading to coupling preferentially to Gs to activate adenylate cyclase and to then increase intracellular cAMP. Therefore, the biological activity of the secretin receptor is determined by examining cAMP accumulation in cells expressing this receptor in response to ligand stimulation (75). Any of the commercially available cAMP measurement kits are useful in this assay.
Acknowledgements
This work was supported by a grant from the National Institutes of Health (DK46577) and by the Mayo Clinic.
4. References
- Bayliss WM, Starling EH. The mechanism of pancreatic secretion. J Physiol 28:325-353, 1902. PMID: 16992627
- Ishihara T, Nakamura S, Kaziro Y, Takahashi T, Takahashi K, Nagata S. Molecular cloning and expression of a cDNA encoding the secretin receptor. Embo J 10:1635-1641, 1991. PMID: 1646711
- Ulrich CD, 2nd, Holtmann M, Miller LJ. Secretin and vasoactive intestinal peptide receptors: members of a unique family of G protein-coupled receptors. Gastroenterology 114:382-397, 1998. PMID: 1646711
- Segre GV, Goldring SR. Receptors for secretin, calcitonin, parathyroid hormone (PTH)/PTH-related peptide, vasoactive intestinal peptide, glucagonlike peptide 1, growth hormone-releasing hormone, and glucagon belong to a newly discovered G-protein-linked receptor family. Trends Endocrinol Metab 4:309-314., 1993. PMID: 18407176
- Foord SM, Bonner TI, Neubig RR, Rosser EM, Pin JP, Davenport AP, Spedding M, Harmar AJ. International Union of Pharmacology. XLVI. G protein-coupled receptor list. Pharmacol Rev 57:279-288, 2005. PMID: 15914470
- Fredriksson R, Lagerstrom MC, Lundin LG, Schioth HB. The G-protein-coupled receptors in the human genome form five main families. Phylogenetic analysis, paralogon groups, and fingerprints. Mol Pharmacol 63:1256-1272, 2003. PMID: 12761335
- Frimurer TM, Bywater RP. Structure of the integral membrane domain of the GLP1 receptor. Proteins 35:375-386, 1999. PMID: 10382665
- Grace CR, Perrin MH, DiGruccio MR, Miller CL, Rivier JE, Vale WW, Riek R. NMR structure and peptide hormone binding site of the first extracellular domain of a type B1 G protein-coupled receptor. Proc Natl Acad Sci USA 101:12836-12841, 2004. PMID: 15326300
- Grace CR, Perrin MH, Gulyas J, Digruccio MR, Cantle JP, Rivier JE, Vale WW, Riek R. Structure of the N-terminal domain of a type B1 G protein-coupled receptor in complex with a peptide ligand. Proc Natl Acad Sci USA 104:4858-4863, 2007. PMID: 17360332
- Koth CM, Abdul-Manan N, Lepre CA, Connolly PJ, Yoo S, Mohanty AK, Lippke JA, Zwahlen J, Coll JT, Doran JD, Garcia-Guzman M, Moore JM. Refolding and characterization of a soluble ectodomain complex of the calcitonin gene-related peptide receptor. Biochemistry 49:1862-1872, 2010. PMID: 20099900
- Parthier C, Kleinschmidt M, Neumann P, Rudolph R, Manhart S, Schlenzig D, Fanghanel J, Rahfeld JU, Demuth HU, Stubbs MT. Crystal structure of the incretin-bound extracellular domain of a G protein-coupled receptor. Proc Natl Acad Sci U S A 104:13942-13947, 2007. PMID: 17715056
- Pioszak AA, Parker NR, Suino-Powell K, Xu HE. Molecular recognition of corticotropin-releasing factor by its G-protein-coupled receptor CRFR1. J Biol Chem 283:32900-32912, 2008. PMID: 1880172
- Pioszak AA, Xu HE. Molecular recognition of parathyroid hormone by its G protein-coupled receptor. Proc Natl Acad Sci USA 105:5034-5039, 2008. PMID: 18375760
- Runge S, Thogersen H, Madsen K, Lau J, Rudolph R. Crystal structure of the ligand-bound glucagon-like peptide-1 receptor extracellular domain. J Biol Chem 283:11340-11347, 2008. PMID: 18287102
- Sun C, Song D, Davis-Taber RA, Barrett LW, Scott VE, Richardson PL, Pereda-Lopez A, Uchic ME, Solomon LR, Lake MR, Walter KA, Hajduk PJ, Olejniczak ET. Solution structure and mutational analysis of pituitary adenylate cyclase-activating polypeptide binding to the extracellular domain of PAC1-RS. Proc Natl Acad Sci USA 104:7875-7880, 2007. PMID: 17470806
- ter Haar E, Koth CM, Abdul-Manan N, Swenson L, Coll JT, Lippke JA, Lepre CA, Garcia-Guzman M, Moore JM. Crystal structure of the ectodomain complex of the CGRP receptor, a class-B GPCR, reveals the site of drug antagonism. Structure 18:1083-1093, 2010. PMID: 20826335
- Underwood CR, Garibay P, Knudsen LB, Hastrup S, Peters GH, Rudolph R, Reedtz-Runge S. Crystal structure of glucagon-like peptide-1 in complex with the extracellular domain of the glucagon-like peptide-1 receptor. J Biol Chem 285:723-730, 2010. PMID: 19861722
- Pang RT, Ng SS, Cheng CH, Holtmann MH, Miller LJ, Chow BK. Role of N-linked glycosylation on the function and expression of the human secretin receptor. Endocrinology 140:5102-5111, 1999. PMID: 10537138
- Ozcelebi F, Holtmann MH, Rentsch RU, Rao R, Miller LJ. Agonist-stimulated phosphorylation of the carboxyl-terminal tail of the secretin receptor. Mol Pharmacol 48:818-824, 1995. PMID: 7476911
- Shetzline MA, Premont RT, Walker JK, Vigna SR, Caron MG. A role for receptor kinases in the regulation of class II G protein-coupled receptors. Phosphorylation and desensitization of the secretin receptor. J Biol Chem 273:6756-6762, 1998. PMID: 9506976
- Holtmann MH, Roettger BF, Pinon DI, Miller LJ. Role of receptor phosphorylation in desensitization and internalization of the secretin receptor. J Biol Chem 271:23566-23571, 1996. PMID: 8798566
- Milligan G. G protein-coupled receptor hetero-dimerization: contribution to pharmacology and function. Br J Pharmacol 158:5-14, 2009. PMID: 19309353
- Milligan G. G protein-coupled receptor dimerization: function and ligand pharmacology. Mol Pharmacol 66:1-7, 2004. PMID: 15213289
- Gao F, Harikumar KG, Dong M, Lam PC, Sexton PM, Christopoulos A, Bordner A, Abagyan R, Miller LJ. Functional importance of a structurally distinct homodimeric complex of the family B G protein-coupled secretin receptor. Mol Pharmacol 76:264-274, 2009. PMID: 19429716
- Harikumar KG, Happs RM, Miller LJ. Dimerization in the absence of higher-order oligomerization of the G protein-coupled secretin receptor. Biochim Biophys Acta 1778:2555-2563, 2008. PMID: 18680717
- Harikumar KG, Pinon DI, Miller LJ. Transmembrane segment IV contributes a functionally important interface for oligomerization of the Class II G protein-coupled secretin receptor. J Biol Chem 282:30363-30372, 2007. PMID: 17726027
- Harikumar KG, Ball AM, Sexton PM, Miller LJ. Importance of lipid-exposed residues in transmembrane segment four for family B calcitonin receptor homo-dimerization. Regul Pept 164:113-119, 2010. PMID: 20541569
- Harikumar KG, Morfis MM, Sexton PM, Miller LJ. Pattern of intra-family hetero-oligomerization involving the G-protein-coupled secretin receptor. J Mol Neurosci 36:279-285, 2008. PMID: 18401761
- Harikumar KG, Simms J, Christopoulos G, Sexton PM, Miller LJ. Molecular basis of association of receptor activity-modifying protein 3 with the family B G protein-coupled secretin receptor. Biochemistry 48:11773-11785, 2009. PMID: 19886671
- McLatchie LM, Fraser NJ, Main MJ, Wise A, Brown J, Thompson N, Solari R, Lee MG, Foord SM. RAMPs regulate the transport and ligand specificity of the calcitonin-receptor-like receptor. Nature 393:333-339, 1998. PMID: 9620797
- Pozvek G, Hilton JM, Quiza M, Houssami S, Sexton PM. Structure/function relationships of calcitonin analogues as agonists, antagonists, or inverse agonists in a constitutively activated receptor cell system. Mol Pharmacol 51:658-665, 1997. PMID: 9106632
- Robberecht P, Conlon TP, Gardner JD. Interaction of porcine vasoactive intestinal peptide with dispersed pancreatic acinar cells from the guinea pig. Structural requirements for effects of vasoactive intestinal peptide and secretin on cellular adenosine 3':5'-monophosphate. J Biol Chem 251:4635-4639, 1976. PMID: 181379
- Turner JT, Jones SB, Bylund DB. A fragment of vasoactive intestinal peptide, VIP(10-28), is an antagonist of VIP in the colon carcinoma cell line, HT29. Peptides 7:849-854, 1986. PMID: 3025826
- Dong M, Miller LJ. Molecular pharmacology of the secretin receptor. Receptors Channels 8:189-200, 2002. PMID: 12529936
- Hacki WH. Secretin. Clin Gastroenterol 9:609-632, 1980. PMID: 7000396
- Chu JY, Yung WH, Chow BK. Secretin: a pleiotrophic hormone. Ann N Y Acad Sci 1070:27-50, 2006. PMID: 16888148
- Yang B, Goulet M, Boismenu R, Ferguson AV. Secretin depolarizes nucleus tractus solitarius neurons through activation of a nonselective cationic conductance. Am J Physiol Regul Integr Comp Physiol 286:R927-934, 2004. PMID: 14715495
- Chu JY, Cheng CY, Lee VH, Chan YS, Chow BK. Secretin and body fluid homeostasis. Kidney Int 79:280-287, 2011. PMID: 20944548
- Siu FK, Lam IP, Chu JY, Chow BK. Signaling mechanisms of secretin receptor. Regul Pept 137:95-104, 2006. PMID: 16930743
- Chu JY, Chung SC, Lam AK, Tam S, Chung SK, Chow BK. Phenotypes developed in secretin receptor-null mice indicated a role for secretin in regulating renal water reabsorption. Mol Cell Biol 27:2499-2511, 2007. PMID: 17283064
- Romano G, Giagu P, Favret G, Bartoli E. Dual effect of secretin on nephron filtration and proximal reabsorption depending on the route of administration. Peptides 21:723-728, 2000. PMID: 10876056
- Trimble ER, Bruzzone R, Biden TJ, Meehan CJ, Andreu D, Merrifield RB. Secretin stimulates cyclic AMP and inositol trisphosphate production in rat pancreatic acinar tissue by two fully independent mechanisms. Proc Natl Acad Sci U S A 84:3146-3150, 1987. PMID: 2437575
- Strader CD, Fong TM, Graziano MP, Tota MR. The family of G-protein-coupled receptors. Faseb J 9:745-754, 1995. PMID: 7601339
- Ostrowski J, Kjelsberg MA, Caron MG, Lefkowitz RJ. Mutagenesis of the beta 2-adrenergic receptor: how structure elucidates function. Annu Rev Pharmacol Toxicol 32:167-183, 1992. PMID: 1318669
- Conner AC, Simms J, Barwell J, Wheatley M, Poyner DR. Ligand binding and activation of the CGRP receptor. Biochem Soc Trans 35:729-732, 2007. PMID: 17635135
- Conner AC, Simms J, Conner MT, Wootten DL, Wheatley M, Poyner DR. Diverse functional motifs within the three intracellular loops of the CGRP1 receptor. Biochemistry 45:12976-12985, 2006. PMID: 17059214
- Huang Z, Chen Y, Pratt S, Chen TH, Bambino T, Nissenson RA, Shoback DM. The N-terminal region of the third intracellular loop of the parathyroid hormone (PTH)/PTH-related peptide receptor is critical for coupling to cAMP and inositol phosphate/Ca2+ signal transduction pathways. J Biol Chem 271:33382-33389, 1996. PMID: 8969199
- Bissonnette BM, Collen MJ, Adachi H, Jensen RT, Gardner JD. Receptors for vasoactive intestinal peptide and secretin on rat pancreatic acini. Am J Physiol 246:G710-717, 1984. PMID: 6204536
- Zhou ZC, Gardner JD, Jensen RT. Receptors for vasoactive intestinal peptide and secretin on guinea pig pancreatic acini. Peptides 8:633-637, 1987. PMID: 2819833
- Korner M, Miller LJ. Alternative splicing of pre-mRNA in cancer: focus on G protein-coupled peptide hormone receptors. Am J Pathol 175:461-472, 2009. PMID: 19574427
- Korner M, Hayes GM, Rehmann R, Zimmermann A, Friess H, Miller LJ, Reubi JC. Secretin receptors in normal and diseased human pancreas: marked reduction of receptor binding in ductal neoplasia. Am J Pathol 167:959-968, 2005. PMID: 16192632
- Korner M, Hayes GM, Rehmann R, Zimmermann A, Scholz A, Wiedenmann B, Miller LJ, Reubi JC. Secretin receptors in the human liver: expression in biliary tract and cholangiocarcinoma, but not in hepatocytes or hepatocellular carcinoma. J Hepatol 45:825-835, 2006. PMID: 16935383
- Korner MU, Hayes GM, Carrigan PE, Rehmann R, Miller LJ, Reubi JC. Wild-type and splice-variant secretin receptors in lung cancer: overexpression in carcinoid tumors and peritumoral lung tissue. Mod Pathol 21:387-395, 2008. PMID: 18223557
- Ding WQ, Kuntz S, Bohmig M, Wiedenmann B, Miller LJ. Dominant negative action of an abnormal secretin receptor arising from mRNA missplicing in a gastrinoma. Gastroenterology 122:500-511, 2002. PMID: 11832464
- Hayes GM, Carrigan PE, Dong M, Reubi JC, Miller LJ. A novel secretin receptor splice variant potentially useful for early diagnosis of pancreatic carcinoma. Gastroenterology 133:853-861, 2007. PMID: 17678920
- Chey WY, Chang TM. Secretin, 100 years later. J Gastroenterol 38:1025-1035, 2003. PMID: 14673718
- Ulrich CD, 2nd, Wood P, Hadac EM, Kopras E, Whitcomb DC, Miller LJ. Cellular distribution of secretin receptor expression in rat pancreas. Am J Physiol 275:G1437-1444, 1998. PMID: 9843782
- Watanabe SI, Chey WY, Lee KY, Chang TM. Release of secretin by licorice extract in dogs. Pancreas 1:449-454, 1986. PMID: 3562439
- Gregory RA. The Bayliss-Starling lecture 1973. The gastrointestinal hormones: a review of recent advances. J Physiol 241:1-32, 1974. PMID: 4609404
- Glaser S, Lam IP, Franchitto A, Gaudio E, Onori P, Chow BK, Wise C, Kopriva S, Venter J, White M, Ueno Y, Dostal D, Carpino G, Mancinelli R, Butler W, Chiasson V, DeMorrow S, Francis H, Alpini G. Knockout of secretin receptor reduces large cholangiocyte hyperplasia in mice with extrahepatic cholestasis induced by bile duct ligation. Hepatology 52:204-214, 2010. PMID: 20578263
- Gardner JD, Jackson MJ. Regulation of amylase release from dispersed pancreatic acinar cells. J Physiol 270:439-454, 1977. PMID: 198531
- Bold RJ, Ishizuka J, Townsend CM, Jr., Thompson JC. Secretin potentiates cholecystokinin-stimulated amylase release by AR4-2J cells via a stimulation of phospholipase C. J Cell Physiol 165:172-176, 1995. PMID: 7559798
- Hisatomi A, Unger RH. Secretin inhibits glucagon in the isolated perfused dog pancreas. Diabetes 32:970-973, 1983. PMID: 6137431
- Kofod H. Secretin N-terminal hexapeptide potentiates insulin release in mouse islets. Regul Pept 15:229-237, 1986. PMID: 3538223
- Kofod H, Hansen B, Lernmark A, Hedeskov CJ. Secretin and its C-terminal hexapeptide potentiates insulin release in mouse islets. Am J Physiol 250:E107-113, 1986. PMID: 3513606
- Dong M, Wang Y, Pinon DI, Hadac EM, Miller LJ. Demonstration of a direct interaction between residue 22 in the carboxyl-terminal half of secretin and the amino-terminal tail of the secretin receptor using photoaffinity labeling. J Biol Chem 274:903-909, 1999. PMID: 9873030
- Chow BK, Cheung KH, Tsang EM, Leung MC, Lee SM, Wong PY. Secretin controls anion secretion in the rat epididymis in an autocrine/paracrine fashion. Biol Reprod 70:1594-1599, 2004. PMID: 14749298
- Nishijima I, Yamagata T, Spencer CM, Weeber EJ, Alekseyenko O, Sweatt JD, Momoi MY, Ito M, Armstrong DL, Nelson DL, Paylor R, Bradley A. Secretin receptor-deficient mice exhibit impaired synaptic plasticity and social behavior. Hum Mol Genet 15:3241-3250, 2006. PMID: 17008357
- Hadac EM, Ghanekar DV, Holicky EL, Pinon DI, Dougherty RW, Miller LJ. Relationship between native and recombinant cholecystokinin receptors: role of differential glycosylation. Pancreas 13:130-139, 1996. PMID: 8829180
- Hsu CH, Yin KW, Hong TH, Tang TK, Lee LS, Chang TH, Liu JD, Chen PH. Preparation of radioiodinated secretin for radioimmunoassay. Ann Nucl Med 5:83-87, 1991. PMID: 1764343
- de Muckadell OB, Fahrenkrug J. Preparation of 125I-labeled synthetic porcine secretin for radioimmunoassay. Scand J Clin Lab Invest 36:661-668, 1976. PMID: 14392
- Kofod H. Synthesis of biologically active porcine secretin and [ITyr10] porcine secretin. Int J Pept Protein Res 37:185-190, 1991. PMID: 1651289
- Dong M, Asmann YW, Zang M, Pinon DI, Miller LJ. Identification of two pairs of spatially approximated residues within the carboxyl terminus of secretin and its receptor. J Biol Chem 275:26032-26039, 2000. PMID: 10859300
- Dong M, Lam PC, Gao F, Hosohata K, Pinon DI, Sexton PM, Abagyan R, Miller LJ. Molecular approximations between residues 21 and 23 of secretin and its receptor: Development of a model for peptide docking with the amino terminus of the secretin receptor. Mol Pharmacol 72:280-290, 2007. PMID: 17475809
- Dong M, Lam PC, Pinon DI, Orry A, Sexton PM, Abagyan R, Miller LJ. Secretin occupies a single protomer of the homodimeric secretin receptor complex: insights from photoaffinity labeling studies using dual sites of covalent attachment. J Biol Chem 285:9919-9931, 2010. PMID: 20100828
- Dong M, Lam PC, Pinon DI, Sexton PM, Abagyan R, Miller LJ. Spatial approximation between secretin residue five and the third extracellular loop of its receptor provides new insight into the molecular basis of natural agonist binding. Mol Pharmacol 74:413-422, 2008. PMID: 18467541
- Dong M, Li Z, Pinon DI, Lybrand TP, Miller LJ. Spatial approximation between the amino terminus of a peptide agonist and the top of the sixth transmembrane segment of the secretin receptor. J Biol Chem 279:2894-2903, 2004. PMID: 14593094
- Dong M, Li Z, Zang M, Pinon DI, Lybrand TP, Miller LJ. Spatial approximation between two residues in the mid-region of secretin and the amino terminus of its receptor. Incorporation of seven sets of such constraints into a three-dimensional model of the agonist-bound secretin receptor. J Biol Chem 278:48300-48312, 2003. PMID: 14500709