Entry Version:
Citation:
Pancreapedia: Exocrine Pancreas Knowledge Base, DOI: 10.3998/panc.2011.36
Attachment | Size |
---|---|
![]() | 579.84 KB |
Gene Symbols: Peptides, Nppa, Nppb, Nppc; Receptors, Npr1, Npr2, Npr3
1. General Structure and Function
In 1981, de Bold and co-workers reported that the administration of an atrial homogenate to rats induced diuresis and natriuresis, thereby establishing for the first time the connection between the heart and the kidney (10). Soon afterwards, the substance with diuretic and natriuretic properties present in atrial extracts was identified as a 28 amino acid peptide containing an intra chain disulfide bond that was termed atrial natriuretic factor (ANF, also referred to as atrial natriuretic peptide [ANP]) (13). This was a hallmark in the physiological field because it advanced the concept that the heart was not only a mechanical pump, but also an endocrine organ. Homologous natriuretic peptides (NPs) were also isolated and characterized: B-type natriuretic peptide (BNP), which shows activities similar to ANF and C-type natriuretic peptide (CNP) which has weak natriuretic and diuretic properties and functions mostly as a paracrine/autacrine factor (57-59). All three members contain the conserved sequence CFGXXXDRIXXXXGLGC where X stands for any amino acid. The flanking cysteines form a 17-amino acid disulfide linked ring that is critical for biological activity (30). The family of NPs plays a relevant role in the regulation of cardiovascular function as well as in sodium and water homeostasis (11). ANF and BNP are predominantly synthesized, stored and released by the heart upon mechanical (atrial stretch) and/or neuroendocrine stimuli (endothelin-1 or -adrenergic stimulation) whereas CNP is mainly produced by endothelial cells and neurons (8,25,57). Later studies revealed the existence of extra-cardiac sites of production for ANF including the brain, gastrointestinal tract, liver, and salivary glands among others (8,16,22). The stimuli promoting the release from these sources still remain elusive but it has been suggested that in the gastrointestinal tract, intestinal distention, analogous to atrial stretch, would stimulate ANF release. Cholinergic and peptidergic neural pathways have also been shown to mediate the release of ANF by the stomach (15). NPs released by extra cardiac sources function as paracrine and/or autacrine factors rather than hormones. Like many peptides, NPs are synthesized as prepropeptides and following signal peptide removal they undergo an additional proteolytic cleavage to render biologically active peptides. They are degraded by extracellular proteases and a receptor-mediated mechanism (40,42). Biochemical, immunohistochemical, and molecular biology studies indicate that NPs and their receptors are quite widespread in their tissue distributions, suggesting pleiotropic actions at both systemic and local levels.
The cellular responsiveness of NPs is manifested through specific cell surface receptors widely spread in different target tissues (38,40). Three single membrane-spanning receptors have been characterized: NPR-A, NPR-B, and NPR-C which display distinct affinities for the members of the family (14,40,60). As NPR-A and NPR-B were first identified as guanylyl cyclase (GC) family members they are also referred to as GC-A and GC-B. ANF preferentially binds to NPR-A and NPR-C whereas CNP binds to NRP-B (24,60). The NPR-C binds all NP with similar affinity and it is the most abundant receptor in all tissues including the digestive system.
NPR-A and NPR-B have a relative molecular mass of approximately 120 kDa and the same overall topology (30,60) (Figure 1). The extracellular binding domains of NPR-A and NPR-B show approximately 44% sequence identity whereas the GC catalytic regions show 88% and the tyrosine kinase domains 60% identity. Although the physiological significance of the tyrosine kinase domain has not yet been established, it has been shown that it binds ATP leading to increased efficacy of the receptor function. Ligand binding to NPR-A and NPR-B activates particulate GC causing a rapid increase in cGMP which in turn activates downstream effectors such as cGMP dependent protein kinases, cGMP gated ion channels and cGMP sensitive phosphodiesterases in order to bring about biological responses (40,42). NPR-A and NPR-B can be phosphorylated on various serines and threonines residues at the amino terminal portion of the kinase homology domain (41). Dephosphorylation of these sites causes receptor desensitization as opposed to G-protein receptors which are desensitized by phosphorylation (41). Gycosylation sites are also present in the extracellular domain and, although such sites are not involved in ligand binding, they are essential for the expression of functional receptors (30,32). It was initially proposed that signaling by NPR-A was mediated by hormone-induced receptor dimerization in a mechanism analogous to those proposed for the growth hormone and cytokine receptors but later it was shown that dimerization itself is not responsible for GC activation (25,30). Most studies indicate that the physiological receptor dimer structure is represented by the “head-to-head” dimer (31). The receptor contains a unique structure near the membrane termed juxtamembrane signaling motif that it is strictly conserved among GC coupled receptors (21). Upon ligand binding the extracellular domain monomers undergo a twisting motion proposed to initiate signaling (31). NPR-A is mostly found in peripheral organs and mediates most of the known actions of ANF. NPR-B is localized mainly in the brain and vascular tissues and mediates the actions of CNP in the central nervous system and the vascular bed (38,40).
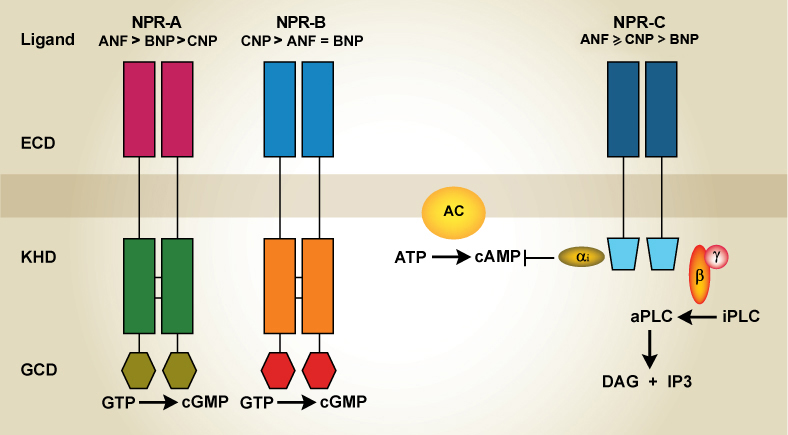
Figure 1: Schematic representation of natriuretic peptide receptors: NPR-A, NPR-B and NPR-C and coupled signaling. ECD: Extracellular domain; KHD: kinase homology domain; GCD: guanylyl cyclase domain; AC: adenylyl cyclase; aPLC: activated phospholipase C; iPLC: inactivated phospholipase C; DAG: diacylglycerol; IP3: inositol triphosphate.
On the other hand, NPR-C receptors are disulphide linked homodimers with a single transmembrane domain, an extracellular domain of approximately 440 amino acids and a short 37 amino acid intracellular tail that bears no homology to any other known receptor protein (3,14,63) (Figure 1). The extracellular domain is about 30% identical to the cyclase linked receptors, it is glycosylated on three Asn (41, 248 and 349) and contains two sets of disulfide bonds between Cys63-Cys91 and Cys168-Cys216 which are conserved in NPR-A and NPR-B (40,56). This receptor subtype is devoid of the GC and tyrosine kinase domains. Given its small intracellular structure NPR-C was initially considered a biological silent receptor involved in the clearance of bound ligands by internalization and degradation (28). However, following its discovery, accumulating evidence supported that several ANF-mediated responses involved intracellular signaling distinct from the cGMP pathway (3). In this regard, we and others showed that ANF stimulates phospholipase C (PLC) (2,6). Later studies showed that the cytoplasmic domain of NPR-C contains several Gi activator sequences characterized by the presence of two basic amino acids at the NH2 terminal and B-B-X-B or B-B-X-X-B at the COOH terminal, where B and X denote basic amino acid and non-basic amino acid, respectively (2,34,37). NPR-C is coupled to a Gi guanine nucleotide regulatory protein(2,18,35). The subunit of Gi inhibits adenylyl cyclase lowering cAMP levels whereas βγ subunits activate PLCβ increasing phosphatidylinositol turnover (44,45,55) (Figure 1). However, NPR-C is not a traditional G-protein coupled receptor in that it is not a heptahelical receptor with seven transmembrane spanning domains as the majority of receptors coupled to inhibitory and stimulatory G proteins.
Actually, NPR-C is considered a disulfide-bridged homodimer of 67 and 77 kDa subunits (4,44,45,55,63,64) where the former is coupled to adenylyl cyclase inhibition and PLC activation whereas the latter is involved in ligand internalization as a clearance receptor (33,35,40,45,55,63).The cellular mechanisms underlying NPR-C-mediated NP internalization and degradation are similar to those of the receptors for low-density lipoproteins. Similar features include lysosomal ligand hydrolysis and recycling of the ligand-free receptor back the plasma membrane (42). Although not demonstrated yet, internalization is believed to occur through a clathrin-dependent mechanism. The receptor subtype signaling through Gi is the predominant NP receptor in visceral and vascular smooth muscle cells. Unfortunately there are no selective antagonists for NP receptors, the only available pharmacological tool is des[Gln18, Ser19, Gly20, Leu21, Gly22]ANP4-23-amide (c-ANP4-23), which is a truncated peptide that selectively binds to NPR-C and displays no affinity for NPR-A or NPR-B (28). This ring deleted analog of ANF has helped to reveal NPR-C function and regulation.
A variety of genetically engineered mice have been generated to study the physiological function of the components of the NP system, but their description is beyond the scope of the present review (23).
2. Specific Function in the Pancreas
The participation of ANF in the regulation of gastrointestinal physiology is supported by the finding that ANF gene expression varies according to feeding and fasting conditions (17). Studies from our laboratory reported that centrally or peripherally applied ANF and CNP exert biological actions mainly through NPR-C activation in the liver, pancreas and salivary glands and NPRA/NPRB in the central nervous system (6,7,47-53,61). Other gastrointestinal effects reported for ANF include regulation of intestinal motility and secretion as well as gastric acid secretion (9,29). We found that centrally applied CNP stimulates pancreatic secretion through vagal pathways and further that it interacts with secretin and cholecystokinin (CCK) in the brain to modify pancreatic function (47). CNP action is not mimicked by c-ANP4-23 supporting that brain GC coupled receptors (NPR-A/NPR-B) mediate the response (47). CNP also stimulates amylase release in isolated pancreatic acini through the activation of NRP-C receptors coupled to PLC activation showing a concentration-dependent biphasic response similar to that elicited by known pancreatic secretagogues (49). Early immunohistochemical studies revealed the presence of immunoreactive ANF in acinar and centroacinar cells as well as cells of the intercalated ducts in the pancreas and nerve fibers (1). It was also reported that pancreatic acini exposed to ANF show a rapid increase in cGMP synthesis but without an effect on the secretory process either basal or stimulated by CCK, secretin or carbachol (19). Later studies from our laboratory in rats showed that the three natriuretic peptide receptors are all present in the exocrine pancreas (49). We found that ANF stimulates pancreatic exocrine secretion in a dose-dependent fashion through NPR-C receptors coupled the phosphoinositide pathway (53). ANF further enhances the secretory response to CCK and secretin supporting an interaction with the major hormones controlling pancreatic function and suggesting that ANF could have a CCK-like effect. ANF increases phosphoinositide hydrolysis but fails to affect basal or forskolin-evoked cAMP levels (53). However it reduces secretin-evoked cAMP content in the pancreas showing that it does not behave like CCK at the signaling level since this hormone does not affect cAMP content stimulated by secretin (50). When the intracellular mechanisms underlying the interaction between ANF and secretin were further studied we found that in isolated pancreatic acini ANF abolishes cAMP accumulation evoked by any dose of secretin (50). However lower doses of ANF (<100nM) dose-dependently reduce EC-50 secretin-evoked cAMP. These findings correlate with in vivo experiments showing that subthreshold doses of ANF potentiate secretin-evoked secretion whereas higher doses blunt secretin secretory response (50). ANP fails to affect cAMP levels stimulated by amthamine (selective H2 agonist) or isoproterenol (β-adrenergic agonist) but it abolishes vasoactive intestinal peptide (VIP)-induced cAMP formation similar to effects on secretin (50). It should be noted that the intracellular amount of cAMP induced by secretin or VIP is considerably higher than that evoked by amthamine or isoproterenol. We found that the mechanisms underlying negative regulation of secretin-evoked signaling by ANF involves cAMP efflux to the extracellular compartment through multidrug resistance proteins (MRPs) (43). Although three members of this family have been identified as ATP-dependent export pumps for cyclic nucleotides, MRP4 shows higher selectivity for cAMP (12,46,54,62). ANF enhances cAMP efflux induced by secretin and VIP through NPR-C receptors coupled to the PLC/PKC pathway (43). However it does not affect amthamine or isoproterenol evoked cAMP which correlates with the finding that cAMP egression is closely related to the amount formed within cells. In time-course studies with active phosphodiesterases, levels of intracellular and extracellular cAMP increase earlier after the addition of secretin and ANF (1 min) than after the addition of secretin alone (3 min) (43). Furthermore, extracellular cAMP is observed earlier in the presence of ANF. Secretin by itself also promotes cAMP efflux, but the mechanism does not involve the PLC/PKC pathway (43). It was proposed that cyclic nucleotide egression operates when phosphodiesterase activity is limited but our findings as well as several studies in other cell types show that the efflux of cAMP occurs in the presence of active phosphodiesterases and appears to be related to a high content of the nucleotide formed which might eventually become harmful to the cell. We found that in the exocrine pancreas MRP4, MRP5 and MRP8 mRNAs are expressed but only MRP4 is detected at the protein level. By the siRNA approach we identified in AR42J cells that MRP-4 mediates secretin-evoked cAMP efflux (43). We found that these cells express the three members of the MRP family involved in cyclic nucleotide egression, MRP4, MRP5 and MRP8. The efflux of cAMP occurs in AR42J cells exposed to secretin and is dramatically reduced in cells expressing MRP4 small interfering RNA, supporting that the efflux is mediated by MRP4 (43). The egression of cAMP by MRPs may represent an additional mechanism to phosphodiesterase action and receptor desensitization to restrict the intracellular accumulation of the cyclic nucleotide within the pancreatic cells. In rats, levels of cAMP in plasma and pancreatic juice increase after infusion with secretin alone, but levels of the cyclic nucleotide are further enhanced in the presence of ANF (43). Figure 2 illustrates the proposed regulation of secretin coupled signaling by ANF through NPR-C receptors in the exocrine pancreas.
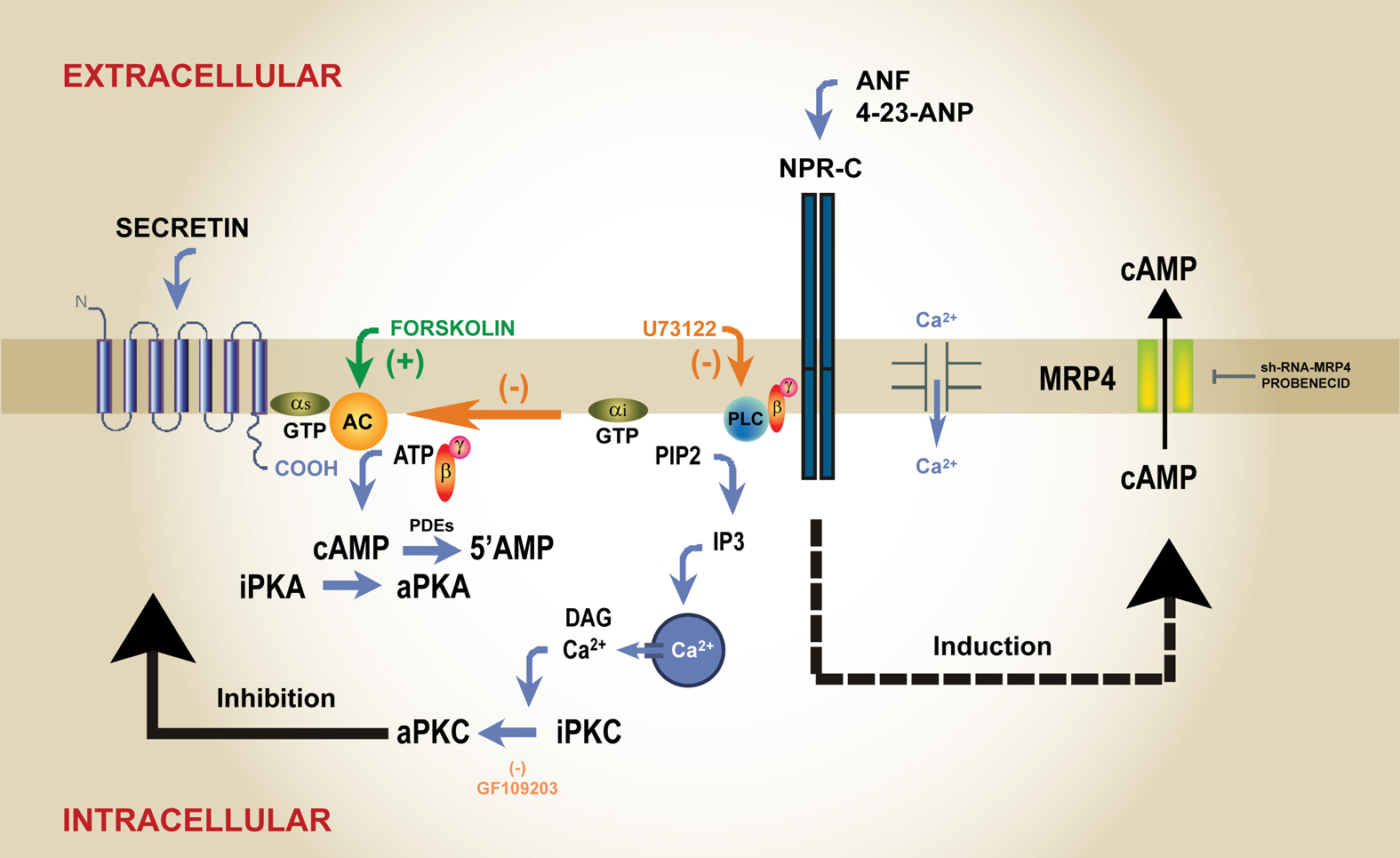
Figure 2: ANF signaling through NPR-C receptor in the exocrine pancreas. AC: adenylyl cyclase; PKA: protein kinase A; PCK: protein kinase C; PLC: phospholipase C; MRP4: multridrug resistance associated protein 4; PDEs: phosphodiesterases; IP3: inositol triphosphate; 4-23 ANP: NRP-C selective agonist; GF109203: PKC inhibitor; U73122: PLC inhibitor; probenecid: general inhibitor of MRPs; forskolin: direct activator of adenylyl cyclase catalytic subunit.
Although egression of cAMP is supported by several studies, the physiological significance of extracellular cAMP still remains to be fully elucidated (5,20). ANF by enhancing cAMP efflux would possibly play a beneficial role in pathophysiological situations like acute pancreatitis provided that it has been shown that cAMP sensitizes pancreatic granules in a context of PLC activation (27,39). Preliminary studies from our laboratory support the protective role of ANF in the early events triggering acute pancreatitis.
3. Tools to Study Natriuretic Peptide System
a. Peptides
Synthetic peptides ANF, CNP and c-ANP4-23 (NPR-C selective agonist) are commercially available from American Peptide (#14-5-4, #14-1-53, and # 14-1-45)
b. Antibodies
Rabbit polyclonal antibody raised against amino acids 141-440 of NPR-C is commercially available from Santa Cruz Biotechnologies (sc-25487).
c. Animal models
Genetically engineered mice have been generated to study NPs and their receptors (23).
Acknowledgments
Our studies were financed by grants from the University of Buenos Aires, the Consejo Nacional de Investigaciones Científicas y Técnicas (CONICET) and the Agencia Nacional de Promoción Científica y Tecnológica (ANPCyT).
4. References
- Adeghate E, Ember Z, Donath T, Pallot DJ, Singh J. Immunohistochemical identification and effects of atrial natriuretic peptide, pancreastatin, leucin-enkephalin and galanin in the porcine pancreas. Peptides, 17: 503-509, 1996. PMID: 8735979
- Anand Srivastava B, Sehl PD, Lowe DG. Cytoplasmic domain of natriuretic peptide receptor C inhibits adenylyl cyclase. Involvement of a persussis toxin sensitive G protein. J Biol Chem., 271: 19324-19329, 1996. PMID: 8702617
- Anand Srivastava MB. Natriuretic peptide receptor C signaling and regulation. Peptides, 26: 1044-1059, 2005. PMID: 15911072
- Arejian M, Li Y, Anand-Srivastava MB. Nitric oxide attenuates the expression of natriuretic peptide receptor C and associated adenylyl cyclase signaling in aortic vascular smooth muscle cells: role of MAPK. Am J Physiol Heart Circ Physiol., 296: H1859-H1867, 2009. PMID: 19252090
- Bankir L, Ahloulay M, Devreotes PN, Parent CA. Extracellular cAMP inhibits proximal reabsorption: are plasma membrane cAMP receptors involved? Am J Physiol Renal Physiol., 282: F376-F392, 2002. PMID: 11832418
- Bianciotti LG, Vatta MS, Elverdin JC, di Carlo MB, Negri G, Fernandez BE. Atrial natriuretic factor induced amylase output in the rat parotid gland appears to be mediated by the inositol phosphate pathway. Biochem Biophys Res Commun., 247: 123-128, 1998. PMID: 9636666
- Bianciotti LG, Vatta MS, Vescina C, Trippodi V, Sabbatini ME, Fernandez BE. Centrally applied atrial natriuretic factor diminishes bile secretion in the rat. Regul Pept., 102: 127-133, 2001. PMID: 11730985
- Cao LH, Yang XL. Natriuretic peptides and their receptors in the central nervous system. Prog Neurobiol., 84: 234-248, 2008. PMID: 18215455
- Cherner JA, Singh G, Naik L. Atrial natriuretic factor activates membrane-bound guanylate cyclase of chief cells. Life Sci., 47: 669-677, 1990. PMID: 1976196
- de Bold AJ, Borenstein HB, Veress AT, Sonnemberg H. A rapid and potent natriuretic response to intravenous injection of atrial myocardial extract in rats. Life Sci., 28: 89-94, 1981. PMID: 7219045
- de Bold AJ, Bruneau BG, de Bold ML. Mechanical and neuroendocrine regulation of the endocrine heart. Cardiovasc Res., 31: 7-18, 1996. PMID: 8849584
- Deeley RG, Westlake C, Cole SPC. Transmembrane transport of endo- and xenobiotics by mammalian ATP-binding cassette multidrug resistance proteins. Physiol Rev., 86: 849-899, 2006. PMID: 16816140
- Flynn TG, de Bold ML, de Bold AJ. The amino acid sequence of an atrial peptide with potent diuretic and natriuretic properties. Biochem Biophys Res Commun., 117: 859-865, 1983. PMID: 6230081
- Fuller F, Porter JG, Arfsten AE, Miller J, Schilling JW, Scarborough RM, Lewicki JA, Schenk DB. Atrial natriuretic peptide clearance receptor. Complete sequence and functional expression of cDNA clones. J Biol Chem., 263: 9395-9401, 1988. PMID: 2837487
- Gower WR Jr, Dietz JR, Mc Cuen RW, Fabri PJ, Lerner EA, Schubert ML. Regulation of atrial natriuretic secretion by cholinergic and PACAP neurons in the gastric antrum. Am J Physiol Gastrointest Liver Physiol., 284:G68-G74, 2003. PMID: 12488235
- Gower WR Jr, Dietz JR, Vesely DL, Finley CL, Scholnick KA, Fabri PJ, Cooper DR, Chalfant CE. Atrial natriuretic peptide gene expression in the rat gastrointestinal tract. Biochem Biophys Res Commun., 202: 562-570, 1994. PMID: 8037763
- Gower WR Jr, Salhab KF, Foulis WL, Pillai N, Bundy JR,Vesely DL, Fabri PJ, Dietz JR. Regulation of atrial natriuretic peptide gene expression in gastric antum by fasting. Am J Physiol Regul Integr Comp Physiol., 278: R770-R780, 2000. PMID: 10712300
- Hashim S, Li Y, Anand-Srivastava MB. Small cytoplasmic domain peptides of natriuretic peptide receptor-C attenuate cell proliferation through Gialpha protein/MAP kinase/PI3-kinase/AKT pathways. Am J Physiol Heart Circ Physiol., 291: H3144-H3153, 2006. PMID: 16920814
- Heisler S, Kopelman H, Guy-Chabot, Morel G. Atrial natriuretic factor and exocrine pancreas: Effects on the secretory process. Pancreas 2: 243-251, 1987. PMID: 2442744
- Hofer AM, Lefkimmiatis K. Extracellular calcium and cAMP: second messengers as “third messengers”? Physiology, 22: 320-327, 2007. PMID: 17928545
- Huo X, Abe T, Misono KS. Ligand binding-dependent limited proteolysis of the atrial natriuretic peptide receptor: juxtamembrane hige structure essential for transmembrane signal transduction. Biochemistry, 38: 16941-16951, 1999. PMID: 10606529
- Janowski M, Petrone C, Tremblay J, Gutkowska J. Natriuretic peptide system in the rat submaxillary gland. Regul Pept., 62: 53-61, 1996. PMID: 8738883
- Kishimoto I, Tokudome T, Nakao K, Kangawa K. Natriuretic peptide system: an overview of studies using genetically engineered animal models. The FEBS J., 278: 1830-1841, 2011. PMID: 21477073
- Koller KJ, Lowe DG, Bennett GL, Minamino N, Kangawa K, Matsuo H, Goeddel DV. Selective activation of the B natriuretic peptide receptor by C-type natriuretic peptide (CNP). Science, 252: 120-123, 1991. PMID: 1672777
- Komatsu Y, Nakao, K, Suga S, Ogawa Y, Mukoyama M, Arai H, Shirakami G, Hosoda K, Nakagawa O, Hama N, Kishimoto I, Imura H. C-type natriuretic peptide (CNP) in rats and humans. Endocrinology, 129: 1104-1116, 1991. PMID: 1855454
- Labrecque J, Mc Nicoll N, Marquis M, De Lean A. A disulfide bridged mutant of natriuretic peptide receptor-A displays constitutive activity. Role of receptor dimerization in signal transduction. J Biol Chem., 274: 9752-9759, 1999. PMID: 10092664
- Lu Z, Kolodenick RH, Karne S, Nyce M, Gorelick F. Effect of ligands that increase cAMP on caerulein-induced zymogen activation in pancreatic acini. Am J Physiol Gastrointest Liver Physiol., 285: G822-G828, 2003. PMID: 12881228
- Maack T, Suzuki M, Almeida FA, Nussenzveig D, Scarborough RM, Mc Enroe GA, Lewicki JA. Physiological role of silent receptors of atrial natriuretic factor. Science, 238: 675-678, 1987. PMID: 2823385
- Matsushita K, Nishida Y, Hosomi H, Tanaka S. Effects of atrial natriuretic peptide on water and NaCl absoption across the intestine. Am J Physiol Regul Integr Comp Physiol., 260: R6-R12, 1991. PMID: 1825159
- Misono KS, Grammer RT, Fukumi H, Inagami T. Rat atrial natriuretic factor: isolation, structure and biological activities of four major peptides. Biochem Biophys Res Commun., 123: 444-451, 1984. PMID: 6541480
- Misono KS, Ogawa H, Qiu Y, Ogata CM. Structural studies of the natriuretic peptide receptor: A novel hormone-induced rotation mechanism for transmembrane signal transduction. Peptides, 26: 957-968, 2005. PMID: 15911065
- Misono KS, Philo JS, Arakawa T., Ogata CM, Qiu Y, Ogawa H, Young HS. Structure, signaling mechanism and regulation of the natriuretic peptide receptor guanylate cyclase. FEBS J., 278: 1818-1829, 2011. PMID: 21375693
- Mouawad R, Li Y, Anand Srivastava MB. Atrial natriuretic peptide C receptor-induced attenuation of adenylyl cyclase signaling activates phosphatidylinositol turnover in A10 vascular smooth muscle cells. Mol Pharmacol., 65: 917-924, 2004. PMID: 15044621
- Murthy KS, Makhlouf GM. Identification of G-protein activating domain of the natriuretic peptide clearance receptor (NPR-C). J Biol Chem., 274: 17587-17592, 1999. PMID: 10364194
- Murthy KS, Teng BQ, Zhou H, Jin JG, Grider JR, Makhlouf GM. G(i-1)/G(i-2) dependent signaling by single transmembrane natriuretic peptide clearance receptor. Am J Physiol Gastrointest Liver Physiol., 278: G974-G980, 2000. PMID: 10859228
- Nishikimi TR, Kiowahara K, Nakao K. Current biochemistry, molecular biology, and clinical relevance of natriuretic peptides. J Cardiol., 57: 131-140, 2011. PMID: 21296556
- Pagano M, Anad Srivastava MB. Cytoplasmatic domain of natriuretic peptide receptor C constitutes Gi activator sequences that inhibit adenylyl cyclase activity. J Biol Chem., 276: 22064-22070, 2001. PMID: 11303026
- Pandey KN. Biology of natriuretic peptides and their receptors. Peptides, 26: 901-932, 2005. PMID: 15911062
- Perides G, Sharma A, Gopal A, Tao X, Dwyer K, Ligon B, Steer ML. Secretin differentially sensitizes rat pancreatic acini to the effects of supramaximal stimulation with caerulein. Am J Physiol Gastrointest Liver Physiol., 289: G713-G721, 2005. PMID: 15920015
- Potter LR, Abbey-Hosch S, Dickey DM. Natriuretic peptides, their receptors, and cyclic guanosine monophosphate-dependent signaling functions. Endocrine Rev., 27: 47-72, 2006. PMID: 16291870
- Potter LR, Hunter T. Phosphorylation of the kinase homology domain is essential for activation of the A-type natriuretic peptide receptor. Mol Cell Biol., 18: 2164-2172, 1998. PMID: 9528788
- Potter LR. Natriuretic peptide metabolism, clearance and degradation. FEBS J., 278:1808-1817, 2011. PMID: 21375692
- Rodriguez MR, Diez F, Ventimiglia MS, Morales V, Copsel S, Vatta MS, Davio CA, Bianciotti LG. Atrial natriuretic factor stimulates efflux of cAMP in rat exocrine pancreas via multidrug resistance associated proteins. Gastroenterology, 140: 1292-1302, 2011. PMID: 21237168
- Rose RA, Giles WR. Natriuretic peptide C receptor signaling in the heart and vasculature. J Physiol., 586: 353-366, 2008. PMID: 18006579
- Rubattu S, Sciarretta S., Morriello A, Calvieri C, Battistoni A, Volpe M. NPR-C: a component of the natriuretic peptide family with implications in human diseases. J Mol Med., 88: 889-897, 2010. PMID: 20563546
- Russel FG, Koenderink JB, Masereeuw R. Multidrug resistance protein 4 (MRP4/ABCC4): a versatile efflux transporter for drugs and signaling molecules. Trends Pharmacol Sci., 29: 200-207, 2008. PMID: 18353444
- Sabbatini ME, Rodriguez MR, Corbo NS, Vatta MS, Bianciotti LG. C-type natriuretic peptide applied to the brain enhances exocrine pancreatic secretion. Eur J Pharmacol., 524: 67-74, 2005. PMID: 16263110
- Sabbatini ME, Rodriguez MR, Dabas P, Vatta MS, Bianciotti LG. C-type natriuretic peptide stimulates pancreatic exocrine secretion in the rat: Role of vagal afferent and efferent pathways. Eur J Pharmacol., 577: 192-202, 2007. PMID: 17900562
- Sabbatini ME, Rodríguez MR, di Carlo MB, Davio CA, Vatta MS, Bianciotti LG. C-type natriuretic peptide enhances amylase release through NPR-C receptors in the exocrine pancreas. Am J Physiol Gastrointest Liver Physiol., 293: G987-G994, 2007. PMID: 17702953
- Sabbatini ME, Vatta MS, Davio CA, Bianciotti LG. Atrial natriuretic factor negatively modulates secretin intracelular signaling in the exocrine pancreas. Am J Physiol Gastrointest Liver Physiol., 292: G349-G357, 2007. PMID: 16973919
- Sabbatini ME, Vatta MS, Vescina C, Castro JL, Fernandez BE, Bianciotti LG. Bile secretion is centrally regulated by C-type natriuretic peptide. Cell Mol Neurobiol., 22: 755-770, 2002. PMID: 12585693
- Sabbatini ME, Vatta MS, Vescina C, Gonzales S, Fernandez BE, Bianciotti LG. NPR-C receptors are involved in C-type natriuretic peptide response on bile secretion. Regul Pept., 15: 13-20, 2003. PMID: 14599710
- Sabbatini ME, Villagra A, Davio CA, Vatta MS, Fernandez BE, Bianciotti LG. Atrial natriuretic factor stimulates exocrine pancreatic secretion in the rat throught NRP-C receptors. Am J Physiol Gastrointest Liver Physiol., 285: G929-G937, 2003. PMID: 12829435
- Sassi Y, Lipskaia L, Vandecasteele G, Nikolaev VO, Hatem SN, Aubart FC, Russel FG, Mougenot N, Vrigdnau C, Lechat P, Lompre AM, Hulot JS. Multidrug resistance associated protein 4 regulates cAMP dependent signaling pathway and controls human and rat SMC proliferation. J Clin Invest., 118: 2747-2757, 2008. PMID: 18636120
- Savoie P, de Champlain J, Anand Srivastava MB. C-type natriuretic peptide and brain natriuretic peptide inhibits adenylyl cyclase activity: interaction with ANF-R2/ANP-C receptors. FEBS Lett., 370: 6-10, 1995. PMID: 7649305
- Stults JT, O´Connell KL, Garcia C, Wong S, Engel AM, Garbes DL, Lowe DG. The disulfide linkages and glycosylation sites on the human natriuretic peptide receptor C-nomodimer. Biochemistry, 33: 11372-11381, 1994. PMID: 7727388
- Suda S, Nakao K, Itoh H, Komatsu Y, Ogawa Y, Hama N, Imura H. Endothelial production of C-type natriuretic peptide and its marked augmentation by transforming growth factor beta. Possible existence of “vascular natriuretic peptide system.” J. Clin Invest., 90: 1145-1149, 1992. PMID: 1522222
- Sudoh T, Kangawa K, Minamino N, Matsuo H. A new natriuretic peptide in the porcine brain. Nature, 332: 78-81, 1988. PMID: 2964562
- Sudoh T, Maekawa K, Kojima M, Minamino N, Kangawa K, Matsuo H. Cloning and sequence analysis cDNA encoding a precursor for human brain natriuretic peptide. Biochem Biophys Res Commun., 159: 1427-1434, 1989. PMID: 2522777
- Tremblay J, Desjardins R, Hum D, Gutkowska J, Hamet P. Biochemistry and physiology of the natriuretic peptide receptor guanylyl cyclases. Mol Cell Biochem., 230: 31-47, 2002. PMID: 11952095
- Ventimiglia MS, Rodríguez MR, Elverdin JC, Davio CA, Vatta MS, Bianciotti LG. Atrial natriuretic factor intracellular signaling in the rat submandibular gland. Regul Pept., 150: 43-49, 2008. PMID: 18455250
- Wielinga PR, van der Heijden I, Reid G, Beijnen JH, Wijnholds J, Borst P. Characterization of MRP4 and MRP5 mediated transport of cyclic nucleotides from intact cells. J Biol Chem., 278: 17664-17671, 2003. PMID: 12637526
- Woodard GE, Xiaohong L, Rosado JA. Characterization of the renal C-type natriuretic peptide receptor in hypertrophied and developing rat kidney. J Mol Endocrinol., 35: 519–530, 2005. PMID: 16326837
- Woodard GE, Li X, Rosado JA. Water deprivation enhances the inhibitory effect of natriuretic peptides on CAMP synthesis in rat adrenal glomeruli. Am J Physiol Renal Physiol., 287: F418-F426, 2004. PMID: 15126246