Entry Version:
Citation:
Pancreapedia: Exocrine Pancreas Knowledge Base, DOI: 10.3998/panc.2014.7
Attachment | Size |
---|---|
![]() | 507.36 KB |
Abstract
Glucagon like peptide-1 (GLP-1) is a GI hormone produced in endocrine cells in the distal small intestine by alternative processing of the proglucagon gene product. In humans it is a 29 amino acid peptide with an amidated carboxyl terminal. It is one of the established incretin hormones as its main action is to promote insulin secretion in response to oral glucose. It has a short half-life in blood of 1.5-3 min due to a high clearance but also activates afferent nerve endings directly before entering the blood. While earlier work focused on direct action on pancreatic beta cells, more recent work has described additional neural actions including a satiety effect. Its action is mediated by a specific G:P-1 receptor. Clinically, GLP-1 mimetics are now used to treat Type 2 diabetes. There have been reports of these drugs inducing pancreatitis and recent suggestions that they may predispose to pancreatic cancer. Overall evidence, however, does not support this causality. The physiological evidence for a direct action of GLP-1 on pancreatic acinar cells is unclear.
1. General Information
The incretin concept arose with the realization that oral glucose induced a greater increase in insulin secretion than did intravenous administration in normal humans (67). Intestinal extracts were then shown to potentiate insulin secretion in dogs and this led to the discovery of two primary gut hormones with incretin activity, gastric inhibitory peptide (GIP) and glucagon like peptide-1 (GLP-1) (9,14,17,25,51,71). The incretin effect accounts for up to 60% of insulin secretion after oral glucose (78). Clinical interest has focused on GLP-1 as GIP is no longer active in Type 2 diabetes. GLP mimetics are currently widely used in the treatment of Type 2 diabetes while both physiological and pathophysiological effects on the exocrine pancreas are less clear.
GLP-1 Synthesis
GLP-1 and GLP-2 were identified in the distal small intestinal mucosa as the product of post-translational processing by prohormone convertase 1/3 (PC1/3) of the proglucagon gene product (8) which in the Islets is processed by PC2 to yield glucagon (71, 82, 92). The human proglucagon amino acid sequence contains 160 amino acids with pancreatic glucagon made up of residues 33-61 and GLP-1 derived from residues 72-108 after further processing (Figure 1).
In gut endocrine cells, proglucagon is processed to glicentin, oxyntomodulin, GLP-1 and GLP-2, all of which have biological activity (25, 51). For GLP-1, the C-terminal amino acid glycine is converted to an amide which increases stability in plasma and the product is known as GLP-1 (1-36 amide). However, prior to secretion the amino terminal is cleaved resulting in GLP-1 (7-36 amide) which is the major form in human plasma and is commonly referred to as GLP-1 (84). This 29 amino acid peptide has about 50 % homology to glucagon and is the primary circulating form in humans which has incretin activity. In some species (rodents, pigs) there are also appreciable amounts of GLP-1 (7-37) which has similar activity to the 7-36 amide. For further details on proglucagon processing see the review by Holst (51). GLP-1 is also produced in the central nervous system where proglucagon is processed similar to the gut (14). In a micellar environment GLP-1 assumes a structure with an N–terminal random coil segment and two helical segments separated by a linker region (104).
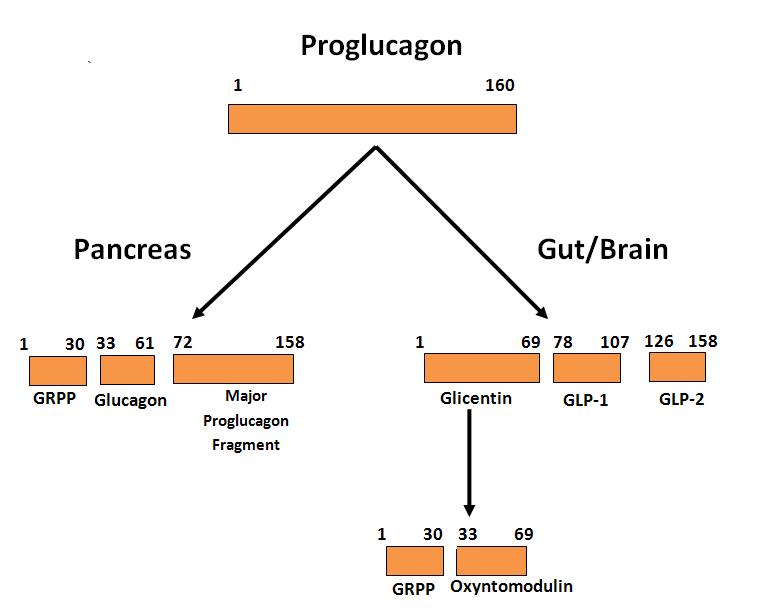
Figure 1. Diagram of the differential processing of the proglucagon gene product in α-cells of the pancreas and L-cells in the gut. Only the biologically active products are shown. For further details of cleavage sites and processing see references (51)
Cells producing and secreting “enteroglucagon” were originally identified by immunohistochemistry with glucagon antibodies and it was noted that the cells in the gut mucosa differed from islet α-cells that produce glucagon (41). These cells were further characterized as containing large (canine) to medium (human) solid round granules by electron microscopy and termed L-cells (10). L-cells are of the open type with apical processes extending towards the gut lumen with secretory granules in the basolateral pole where they can by released and their secretory material enter capillaries. About half of L-cells show costaining for PYY, another GI hormone, which is present in the same secretory granules (80). In the upper gut, GLP-1 can colocalize with CCK (44) or GIP (73). Both immunoreactive gut glucagon and L-cells are present most abundantly in the distal small intestine and colon (29, 58). This distal location presents problems explaining the rapid release after a meal.
GLP-1 Secretion
While GLP-1 secretion was originally described in response to glucose, it also occurs with ingestion of a mixed meal and the GLP-1 response is greater than to glucose alone indicating synergy in the stimulation by fat and protein along with glucose (1, 120). This is also the case for plasma insulin levels in humans where a mixed liquid meal is a stronger stimulus than oral glucose alone. Recent studies of isolated L-cells (90) and a L-cell derived cell line, GLUTag cells, have shown stimulation of GLP-1 secretion by glucose acting to inhibit K+ channels as in islet beta cells, by activation of the SGLT-1 transporter leading to depolarization (86), or activation of sweet taste receptors (T1R2 / T1R3) which activate Gq and PLC. Free fatty acids act on receptors including GPR 43, GPR119 and GPR 120, and FFAR (27, 50, 105), and proteins may act through the Ca2+ sensing receptor (CaSR) which responds to amino acids (21) or the peptide transporter PepT1 (65). Nutrient stimulation causes an intracellular increase in both cAMP and Ca2+ which then mediates GLP-1 secretion (106). The increase in Ca2+ is mediated by IP3 induced release of intracellular Ca2+ and by depolarization leading to activation of voltage dependent Ca2+ channels. A summary diagram is shown in Figure 2.
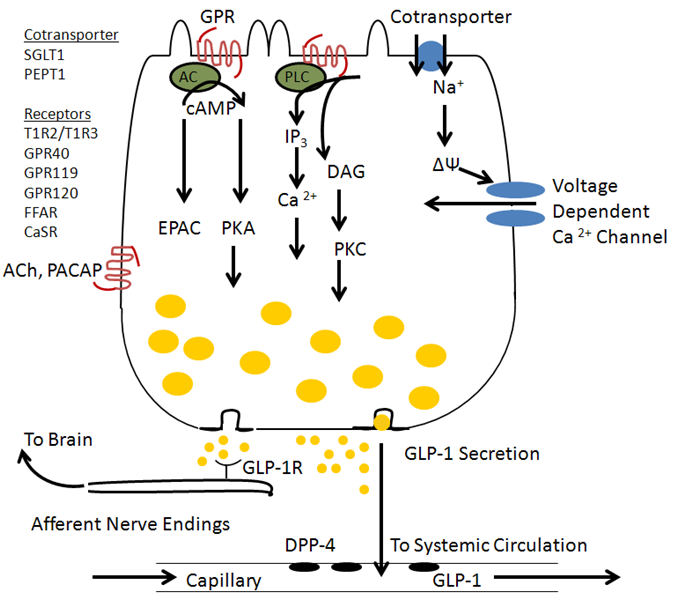
Figure 2. Regulation of GLP-1 secretion by nutrients acting via G protein coupled receptors and transporters. Nutrient receptors are located on the apical surface where exposed to luminal contents and secretion occurs at the basolateral pole where secreted GLP-1 can activate receptors on vagal afferent nerve endings or enter capillaries and travel through the systemic circulation to reach the islets. GLP-1 secretion may also be regulated by neurotransmitters such as ACh and PACAP.
GLP-1 in plasma can be measured by RIA although some antibodies react with multiple proglucagon products containing the antigenic epitope. The most specific antibodies are directed at the amidated C-terminus of GLP-1. The rise in plasma GLP-1 is clearly meal related with fasting levels of about 5-15 pM and stimulated levels of 40-80 pM in normal men (55, 85). The response begins within 10 min but is generally slower than cephalic phase events and peaks after an hour. Ileal instillation of carbohydrate or lipid induced a more rapid stimulation of GLP-1 (59). How GLP-1 secretion occurs so rapidly is still unclear but may be due to the presence of some L-cells in the upper jejunum (29). The vagal nerve may also be involved (91) and somatostatin exerts a strong inhibitory influence (46).
GLP-1 has a plasma half-life of only 1.5-3 min with a high clearance indicating degradation in blood (52, 68). The main enzyme involved, dipeptidyl peptidase-4 (DPP-4) also known as CD26, cleaves off the two N-terminal residues resulting in GLP-1 (9-36 amide) which is inactive or acts as an inhibitor at the GLP-1 receptor (18). This form has a half-life of about 4 min as it is further degraded by the kidneys. DPP-4 is present on the apical endothelial surface of capillaries including those in the intestine. As a result much of the GLP-1 in intestinal veins is already degraded (45). Mice in which CD26 has been deleted showed increased levels of plasma GLP-1 (63). In humans, the half life is similar in healthy subjects and obese patients with type 2 diabetes (110). Because of the rapid degradation of GLP-1, therapeutic use of GLP-1 to enhance insulin secretion makes use of degradation resistant analogs of GLP-1 or DPP-4 inhibitors. The exendins are bioactive peptides isolated from the venom of Gila Monster. Exendin-4 is a 39 amino acid peptide with 53% identity to GLP-1 (32) and is fully active at GLP-1 receptors and not degraded by DPP-4. Exendin-4 (Exenatide) mimicks GLP-1 but is much more potent in vivo following parenteral administration (25). Its half life is about 6 hours and it is given twice daily. A long acting DPP-4 resistant, fatty acylated human GLP-1 analog (Liraglutide) has also been developed and needs to be administered only once daily. DPP-4 inhibitors (DPP-4i) enhance plasma levels of GLP-1 and several other GI hormones including GIP by inhibiting their degradation. A major advantage is that they can be given orally while a potential disadvantage is that other hormones could also be affected. Interestingly, DPP-4 inhibition does not have to take place systemically but only in the intestine as shown with oral administration of low doses of inhibitor (112).
GLP-1 Action
The major target tissues for GLP-1 are the pancreatic islet cells but it also has actions on gastrointestinal secretion and motility of the stomach and pancreas. GLP-1 also affects food intake, body weight, and has direct effects on the heart (51, 97).
GLP-1 exerts its actions on target cells by binding to specific high affinity receptors. These were initially identified by high affinity binding of 125I-GLP or 125I-[Y39]exendin-4 to beta cell lines, gastric chief and parietal cells and pancreatic acinar cells (38, 83, 89, 98). GLP-1 and exendin-4 show a similar nM affinity while glucagon, secretin, VIP and GLP-2 have at least a hundred times lower affinity for the GLP-1 receptor. The rat receptor was cloned by expression cloning of an islet cDNA library followed by identification of human clones (22, 40, 103). The receptor belongs to the G-protein coupled 7 transmembrane domain family and has 40% homology to the secretin receptor. It is considered to belong to the Class B family of GPCRs whose members possess an extracellular N-terminal domain of 100-150 amino acids connected to the 7TM domain core typical of all G protein receptors (23). This N-terminal domain has a characteristic structure and participates in binding of the ligand (2, 69). It also has three glycosylation sites necessary for insertion into the plasma membrane (16, 39).
GLP-1R mRNA is most abundant in islets and lung with lesser amounts in the stomach. In islets, receptors are present on beta cells; whether they are present on alpha or delta cells is controversial (48, 83). The specificity of antibodies to GLP-1R protein is also controversial. The binding properties of the expressed receptor are similar to native receptors. Both native and cloned receptors signal through the activation of both adenylyl cyclase and phospholipase C (72,118). Activation of specific heterotrimeric G proteins involves different regions of the receptors intracellular loops, primarily the third intracellular loop (7, 49, 64). Cyclic AMP, however, is the main intracellular mediator of GLP-1 action in beta cells and other target cell types (24, 61).
2. Action of GLP-1 on the pancreas
Physiology of GLP-1 as an incretin
The primary action of GLP-1 for lowering blood glucose is the stimulation of insulin secretion by potentiating the action of glucose (70, 75, 115). Intestinal release of GLP-1 augments initial insulin secretion in vivo in part through a vagal-vagal reflex leading to cholinergic stimulation of islet beta cells. The direct action of GLP-1 which can also be demonstrated in the perfused pancreas, with isolated islets and with beta cell derived cell lines involves activation of the beta cell GLP-1R which couples through the heterotrimeric G-protein Gs to activate adenylyl cyclase and increase intracellular cAMP (24, 61). Cyclic AMP acts in beta cells through both PKA and Epac2. This action leads to closure of glucose-dependent ATP-sensitive K+ channels which depolarizes the cell and thereby opens voltage dependent Ca2+ channels allowing Ca2+ influx which stimulates insulin secretion. The Epac2 arm activates PLC epsilon through Rap1 and this also leads to increased intracellular Ca2+ (Figure 3). These mechanisms are central to restoring function in type 2 diabetes as in that condition glucose does not stimulate adequate Ca2+ influx. The action is self limiting as when the increased insulin release reduces plasma glucose, the ability of GLP-1 to act is turned off (43). Thus there is little risk of hypoglycemia. In addition to this mechanism, PKA has recently been shown to phosphorylate Snapin and regulate the SNARE complex thereby augmenting Ca2+ mediated exocytosis (99). GLP-1 has also been reported to increase secretory granules docked at the plasma membrane and also increases the frequency of compound exocytosis (56).
In rodent models, GLP-1 also enhances modest beta cell mitogenesis and inhibits apoptosis thereby leading to enhanced beta cell mass (12, 100, 123). There are also reports of enhanced islet neogenesis from duct precursors (19). These actions lead to a prolonged or durable action after ceasing GLP-1 treatment (119). Whether these actions occur in humans is unclear especially since cessation of GLP-1 therapy rapidly leads to the reappearance of diabetes. However, one report indicated that stimulation of freshly isolated human islets inhibited apoptosis (35).
Other actions of GLP-1 that also contribute to restoring glucose regulation in Type 2 diabetes include inhibition of glucagon secretion, slowing of gastric emptying, suppression of appetite and modest reduction in body weight (36, 47, 79, 109). These appear to be less important, however, as restoring GLP-1R in the pancreas in a receptor KO mouse restored glucose tolerance (57).
Glucagon Like Peptide 1 (GLP-1) mimetic drugs which mimick the action of native GLP-1 as an incretin hormone have become a common second line of therapy for Type 2 diabetes (75). Two classes exist. The first, degradation resistant analogs of GLP-1 (Exenatide and Liraglutide) must be given by subcutaneous injection once or twice daily. The second class is inhibitors of the GLP-1 degrading enzyme, dipeptidyl pepitidase IV (Sitagliptin and related drugs) which can be given orally and increase the plasma level of GLP-1 and other peptides degraded by DPP-4. These drugs by increasing insulin secretion and possibly, beta cell mass, decrease plasma glucose and lipids and have a secondary action to reduce food intake and bring about weight loss. Slowing of gastric emptying also plays a role in the action of Exenatide (15). They are often used in combination with a Metformin drug. However, a number of case reports and analysis of adverse effect databases raise the possibility that the drugs increase the incidence of acute pancreatitis. The FDA has issued warnings which have also been contested. For further coverage of this issue see the following references (5, 28, 34, 94, 95). More recently, the possibility that GLP-1 mimetics predispose to pancreatic cancer has been raised and disputed (37, 77).
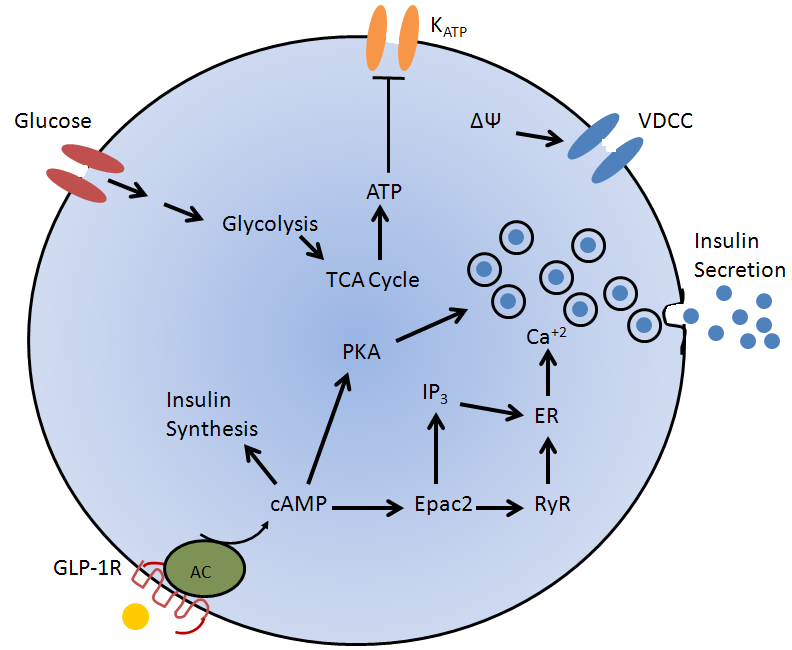
Figure 3. Actions of GLP-1 to potentiate glucose stimulated insulin secretion from islet β cells. GLP-1 binds to specific receptors on beta cells that activate adenylyl cyclase and through cAMP activates PKA and EPAC2 which act to potentiate insulin secretion.
Actions of GLP-1 on exocrine pancreas
Infusion of GLP-1 to mimic postprandial levels inhibits pancreatic exocrine secretion in response to intragastric infusion of a liquid meal in man (117). Although GLP-1 can inhibit gastric emptying thereby reducing pancreatic stimulation, in followup studies, GLP-1 was shown to inhibit pancreatic digestive enzyme secretion stimulated by carbachol infusion (42). This effect appears to explain the carbohydrate stimulated “ileal brake” where plasma GLP-1 increases without a change in PYY (60). This effect may be mediated through central neural pathways as has been established for the action of GLP-1 to inhibit gastric acid secretion (51). Studies in the pig showed GLP-1 inhibited pancreatic secretion induced by hypoglycemia (central neural stimulation) but not that due to vagal nerve stimulation (116). These inhibitory effects of GLP-1 could be mediated by activation of vagal afferents in the distal small intestine or by hormonal effects on the NTS-DMV circuitry (113). It must involve either an action of an inhibitory neurotransmitter or reduction of a stimulatory one.
Direct effects on isolated pancreatic cells
Study of possible direct effects of GLP-1 on acini began with the discovery of biologically active peptides in Gila monstor venom (31,32) and the identification of a specific receptor for Exendin-4 on guinea pig isolated pancreatic acini that increased the production of cAMP (33, 88). This effect was then shown to be mimicked by GLP-1 that acted on the same receptor (89). Interestingly, neither exendin-4 or GLP-1 stimulated amylase release from isolated acini of guinea pig or rat pancreas but both potentiated secretion induced by CCK (62, 89, 114). This potentiation was also seen with other Ca2+ mediated secretagogues. While the possibility exists that these effects were mediated by another receptor such as for VIP or secretin, the weight of the evidence is most consistent for a specific receptor on these acinar cells. However, the failure of GLP-1 to stimulate amylase release in guinea pig acini is surprising as all other ways of increasing cAMP in these cells does stimulate amylase release. Studies with Western blotting and immunohistochemistry to evaluate the presence of GLP-1R on acinar and duct cells in various species are difficult to interpret due to lack of antibody specificity (26, 87). Likewise, PCR studies to date have not given a clear answer as to whether and where a specific GLP-1 receptor exists on exocrine pancreatic cells. In situ hybridization initially revealed pancreatic GLP-1R mRNA only in the β cells (11, 53,107). Reports of localization of GLP-1R to pancreatic ducts have appeared and exendin 4 treatment of isolated human ducts increased the number of insulin positive cells (121). More convincingly, immunoblotting and PCR showed that GLP-1 receptors are present on AR42J cells, a rat pancreatic acinar-derived cell line but which shows some islet characteristics. GLP-1 increased cAMP but did not affect amylase secretion; however, in this cell line GLP-1 increased intracellular Ca2+ levels (124). A recent study reported GLP-1 receptors on activated pancreatic stellate cells both in pancreatitis and isolated stellate cells in culture (76).Overall, the possibility of direct effects on exocrine pancreas is unclear and worthy of further study.
Possible relation to pancreatitis or pancreatic adenocarcinoma
The third type of study of the exocrine pancreas has been to give GLP-1 mimetics or DPP-4 inhibitors to animals over a prolonged period of time and evaluate the pancreas for evidence of pancreatitis or precancerous lesions. Six studies have evaluated the effects of the mimetics, exenatide (81, 101, 102, 122) or liraglutide (74, 102). None of the studies resulted in frank pancreatitis but two reported some increase in pancreatic inflammation at high levels of exenatide. Nachnani et al (74) in a study in rats reported animals receiving exenatide for 75 days showed more acinar inflammation and pyknotic nuclei and these animals weighed less. In a later study of small numbers of rats studied for 10 weeks, exenatide increased inflammatory cell infiltrate with interstitial edema and fibrosis in 5 of 15 rats (122). Two studies evaluated the effect of exendin on pancreatic gene expression in mice. Koehler showed that exendin administration induced antiinflamatory genes such as PAP (54). De Leon compared genes induced by exendin to those expressed following partial pancreatectomy and found a set that included multiple Reg genes as well as fragilis and serpin b1a (20) . Four published studies showed no pancreatitis effects including one in which the pancreas of rats, mice and monkeys showed no change with liraglutide at concentrations 60 fold higher than used in humans for up to two years (81). Other studies showed no effect of exenatide for 13 weeks (101, 102) or liraglutide for 13 weeks (111). Fewer studies have been carried out with DPP-4i. One study reported one rat out of 8 expressing human islet amyloid polypeptide (HIP) as a model for diabetes developed pancreatitis when treated with sitaglyptin for 12 weeks (66). In a larger study of mice expressing HIP, no evidence of pancreatitis was seen in mice given sitaglyptin for 1 year (3). Similar unpublished preclinical data on file at Merck has been summarized (34). Two studies have also evaluated whether exenatide would modify experimental pancreatitis induced by caerulein. No effect was seen by Koehler et al (54) and in the work of Tatarkiewciz et al (101) high doses of exenatide inhibited the pancreatitis. A recent double blind placebo controlled large trial of sitaglyptin for effects on heart disease in humans also showed no effect on the rate of acute or chronic pancreatitis (95).
Other studies have reported effects on overall growth of the pancreas (as opposed to islet growth) or evidence of increased exocrine mitogenesis. Ellenbroek et al (30) reported pancreatic weight was increased after 6 weeks on liraglutide with a 65% increase in proliferating acinar cells and no change in duct cell proliferation. Tschen et al reported increased proliferation of duct cells in response to exendin similar to that found in beta cells (108) and Koehler et al (54) reported exendin increased pancreatic mass in mice after one week. However, most studies of pancreatic mass or exocrine cellular proliferation have reported no change with Exenatide or liraglutide (81, 101, 102, 111). Thus there does not appear to be a consistent change. Other studies have evaluated acinar-ductal metaplasia (ADM) and PanIN formation, a precancerous lesion. In eight rats with transgenic expression of HIP to induce diabetes, three given sitaglyptin showed clear ADM (66). Increased Ki67 staining indicating DNA replication occurred in both ADM and in normal ductal epithelial cells. Gier et al (37) reported PanIN lesions located primarily in pancreatic duct glands of normal rats treated with exendin-4. This is of concern since a recent study of human pancreas from organ donors who had been treated with incretin mimetic drugs for a year also showed the increased presence of PanIN lesions (13). In a study of transgenic mice with a Kras G12D mutation which induces PanINs and eventually pancreatic cancer, treatment with exendin-4 increased higher grade PanIN lesions (37). Further longer term studies of normal and genetically modified rodents are clearly warranted.
3. Tools for the study of GLP-1
a. Antibodies
A large number of antibodies are listed in Biocompare but I have no personal experience with them.
b. Measurement of GLP-1
A number of ELISA or other sandwich antibody based techniques are available in kit form, including from EMD Millipore and ALPCO which measure active GLP-1 without significant contribution from GLP-1 (9-36 amide). These are designed for human plasma and require 100 µl. Eagle Bioscience sells a kit optimized for rat/mouse that requires 20 µl of plasma. As the amino acid sequence is identical between human and laboratory animal species any kit can be used for any species.
c. Mouse Models
Because GLP-1 cannot be deleted specifically without affecting the other products of the proglucagon gene including glucagon, attention has focused on the GLP-1 receptor gene which can be deleted independent of the glucagon receptor. Earlier studies using these mice include (4, 6, 96).
Acknowledgement
We thank Ernesto Bernal-Mizrachi for reading the manuscript and commenting on the use of the GLP-1 mimetics in treating Type 2 diabetes.
4. References
- Ahlkvist, L, Vikman J, Pacini G, Ahren B. Synergism by individual macronutrients explains the marked early GLP-1 and islet hormone responses to mixed meal challenge in mice. Regulatory Peptides 178:29-35, 2012. PMID: 22750278
- Al-Sabah S, Donnelly D. A Model for receptor-peptide binding at the glucagon-like peptide-1 (GLP-1) receptor through the analysis of truncated ligands and receptors. British J of Pharm 140: 339-346, 2003. PMID: 12970080
- Asthon-Mourney K, Subramanian S.L, Zraika S, Samarasekera T, Meier D.T, Goldstein L.C, Hull R.L. One year of sitagliptin treatment protects against islet amyloid-associated β-cell loss and does not include pancreatitis or pancreatic neoplasia in mice. Am J Physiol Endocrinol Metab 305: 475-484, 2013. PMID: 23736544
- Ayala J.E, Bracy D.P, James F.D, Julien B.M, Wasserman D.H, Drucker D.J. The glucagon-like peptide-1 receptor regulates endogenous glucose production and muscle glucose uptake independent of its incretin action. Endocrinology 150: 1155-1164, 2009. PMID: 19008308
- Ayoub W.A, Kumar A.A, Naguib H.S, Taylor H.C. Exenatide-induced acute pancreatitis. Endocr Pract 16: 80-83, 2010. PMID: 19703814
- Baggio L, Kieffer TJ, Drucker D.J. Glucagon-Like Peptide-1, But Not Glucose-Dependent Insulinotropic Peptide, Regulates Fasting Glycemia and Nonenteral glucose Clearance in Mice. Endocrinology 141: 3703-3709, 2000. PMID: 11014225
- Bavec A, Hallbrink M, Langel U, Zorko M. Different role of intracellular lops of glucagon-like peptide-1 receptor in G-protein coupling. Regul Pept 111: 1-3, 2003. PMID: 12609761
- Bell GI, Sanchez-Pescador R, Laybourn PJ, Najarian RC. Exon duplication and divergence in the human preproglucagon gene. Nature 304: 368-371, 1983. PMID: 6877358
- Brubaker PL. Minireview: Update on Incretin Biology: Focus on Glucagon-Like Peptide-1. Endocrinology 151: 1984-1989, 2010. PMID: 20305008
- Buffa R, Capella C, Fontana P, Usellini L, Solcia E. Types of Endocrine Cells in the Human Colon and Rectum. Cell Tiss Res 192: 227-240, 1978. PMID: 699014
- Bullock B.P, Heller R.S, Habener J.F. Tissue distribution of messenger ribonucleic acid encoding the rat glucagon-like peptide-1 receptor. Endocrinology 137: 2968-2978, 1996. PMID: 8770921
- Buteau J, Foisy S, Joly E, Prentiki M. Glucagon-Like Peptide 1 Induces Pancreatic β-Cell Proliferation Via Transactivation of the Epidermal Growth Factor Receptor. Diabetes 52: 124-132, 2003. PMID: 12502502
- Butler A.E, Campbell-Thompson M, Gurlo T, Dawson D.W, Atkinson M, Butler P.C. Marked expansion of exocrine and endocrine pancreas with incretin therapy in humans with increased exocrine pancreas dysplasia and the potential for glucagon-producing neuroendocrine tumors. Diabetes 62: 2595-2604, 2013. PMID: 23524641
- Campbell J.E, Drucker D.J. Pharmacology, physiology, and mechanisms of incretin hormone action. Cell Metabolism 17:819837, 2013. PMID: 23684623
- Cervera A, Wajcberg E, Sriwijitkamol A, Fernandez M, Zuo P, Triplitt C, Musi N, DeFronzo R.A, Cersosimo E. Mechanism of action of exenatide to reduce postprandial hyperglycemia in type 2 diabetes. Am J Physiol Endocrinol Metab 294: E846-E852, 2008. PMID: 18334612
- Chen Q, Miller L.J, Dong M. Role of N-linked glycosylation in biosynthesis, trafficking, and function of the human glucagon-like peptide 1 receptor. Am J Physiol Endocrinol Metab 299: E62-E68, 2010. PMID: 20407008
- Cho Y, Fujita Y, Kieffer T. Glucagon-Like Peptide-1: Glucose Homeostasis and Beyond. Annu. Rev. Physiol. 76: 535-559, 2014. PMID: 24245943
- De Leon D.D, Deng S, Madani R, Ahima R.S, Drucker D.J, Stoffers D.A. Role of Endogenous Glucagon-Like Peptide-1 in Islet Regeneration After Partial Pancreatectomy. Diabetes 52: 365-371, 2003. PMID: 12540609
- De Leon D.D, Farzard C, Crutchlow M.F, Brestelli J, Tobias J, Kaestner K.H, Stoffers D.A. Identification of transcriptional targets during pancreatic growth after partial pancreatectomy and exendin-4 treatment. Physiol Genomics 24: 133-143, 2006. PMID: 16410542
- Deacon C.F, Johnsen A.H, Holst J.J. Degradation of glucagon-like peptide-1 by human plasma in vitro yields an N-terminally truncated peptide that is a major endogenous metabolite in vivo. J Clin Endocrinol Metab 80: 952-957, 1995. PMID: 7883856
- Diakogiannaki E, Pais R, Tolhurst G, Parker H.E, Horscroft J, Rauscher B, Zietek T, Daniel H, Gribble F.M, Reimann F. Oligopeptides stimulate glucagon-like peptides-1 secretion in mice through proton-coupled uptake and the calcium-sensing receptor. Diabetologia, 2013. PMID: 24045836
- Dillon J.S, Tanizawa Y, Wheeler M.B, Leng X.H, Ligon B.B, Rabin D.U, Yoo-Warren H, Permutt M.A, Boyd III A.E. Cloning and functional expression of the human glucagon-like peptide-1 (GLP-1) receptor. J Endocrinol, 133: 1907-1910, 1993. PMID: 8404634
- Donnelly D. The structure and function of the glucagon-like peptide-1 receptor and its ligands. Brit. J. Pharm. 166: 27-41, 2012. PMID: 21950636
- Doyle M.E, Egan J.M. Mechanisms of action of glucagon-like peptide 1 in the pancreas. Pharmacology & Therapeutics 113: 546-593, 2007. PMID:17306374
- Drucker D.J. Incretin Action in the Pancreas: Potential Promise, Possible Perils, and Pathological pitfalls. Diabetes 62: 3316-3323, 2013. PMID: 23818527
- Drucker D.J. The biology of incretin hormones. Cell Metabolism 3: 153-165, 2006. PMID: 16517403
- Edfalk S, Steneberg P, Edlund H. Gpr40 is expressed in enteroendocrine cells and mediates free fatty acid stimulation of incretin secretion. Diabetes 57: 2280-2287, 2008. PMID: 18519800
- Egan A.G, Blind E, Dunder K, de Graeff P.A, Hummer B.T, Bourcier T, Rosebraugh C. Pancreatic safety of incretin-based drugs – FDA and EMA assessment. N Engl J Med 370:794-797, 2014. PMID: 24571751
- Eissele R, Goke R, Willemer S, Harthus H.P, Vermeer H, Arnold R, Goke B. Glucagon-like peptide-1 cells in the gastrointestinal tract and pancreas of rat, pig and man. Eur J Clin Invest 22: 283-291, 1992. PMID: 1499644
- Ellenbroek J.H, Tons H.A, Westerouen van Meeteren M.J, de Graaf N, Hanegraaf M.A, Rabelink T.J, Carlotti F, de Koning E.J. Glucagon-like peptide-1 receptor agonist treatment reduces beta cell mass in normoglycaemic mice. Diabetologia 56: 1980-1986, 2013. PMID: 23771206
- Eng J, Andrews PC, Kleinman WA, Singh L, Raufman JP. Purification and structure of Exendin-3, a new pancreatic secretagogue isolated from Heloderma horridum venom. J Biol Chem, 265:20259-20262, 1990.
- Eng J, Kleinman W, Singh L, Singh G, Raufman J.P. Isolation and Characterization of Exendin-4, an Exendin-3 Analogue, from Heloderma suspectum Venom. J Biol chem. 267: 7402-7405, 1992. PMID: 1313797
- Eng J, Raufman J.P, Singh G, Singh L. Truncated Glucagon-like Peptide-1 Interacts with Exendin Receptors on Dispersed Acini from Guinea Pig Pancreas. J Biol Chem, 267: 21432-21437, 1992. PMID: 1328231
- Engel S.S, Williams-Herman D.E, Golm G.T, Clay R.J, Machotka S.V, Kaufman K.D, Goldstein B.J. Sitagliptin: review of preclinical and clinical data regarding incidence of pancreatitis. Int J Clin Pract 64: 984-990, 2010. PMID: 20412332
- Farilla L, Bulotta A, Hirshberg G, Li-Calzi S, Khoury N, Noushmehr H, Bertolotto C, Di Mario U, Harlan D.M, Perfetti R. Glucagon-like peptide 1 inhibits cell apoptosis and improves glucose responsiveness of freshly isolated human islets. Endocrinology 144: 5149-5158, 2003. PMID: 12960095
- Flint A, Raben A, Astrup A, Holst J.J. Glucagon-like Peptide 1 Promotes Satiety and Suppresses Energy Intake in Humans. J Clin Invest 101: 515-520, 1998. PMID: 9449682
- Gier B, Matveyenko A.V, Kirakossian D, Dawson D, Dry S.M, Butler P.C. Chronic GLP-1 Receptor Activation by Exendin-4 Induces Expansion of Pancreatic Duct Glands in Rats and Accelerates Formation of Dysplastic Lesions and Chronic Pancreatitis in the KrasG12D Mouse Model. Diabetes 61: 1250-1262, 2012. PMID: 22266668
- Goke R, Conlon J.M. Receptors for glucagon-like peptide-1(7-36) amide on rat insulinoma-derived cells. J. Endocr. 116: 357-362, 1988. PMID: 2832504
- Goke R, Just R, Lankat-Buttgereit B, Goke B. Glycosylation of the GLP-1 receptor is a prerequisite for regular receptor function. Peptides 15: 675-681, 1994. PMID: 7937345
- Grazianno M.P, Hey P.J, Borkowski D, Chicchi G.G, Strader C.D. Cloning and Functional Expression of a Human Glucagon-Like Peptide-1 Receptor. Biochem Biophys Res Commun, 196:141-146, 1993. PMID: 8216285
- Grimelius L, Capella C, Buffa R, Polak J.M, Pearse A.G, Solcia E. Cytochemical and ultrastructural differentiation of enteroglucagon and pancreatic-type glucagon cells of the gastrointestinal tract. Virchows Arch B Cell Pathol 20: 217-228, 1976. PMID: 818803
- Gröger G, Unger A, Holst J.J, Goebell H, Layer P. Ileal Carbohydrates Inhibit Cholinergically Stimulated Exocrine Pancreatic secretion in Humans. Pancretology 22: 23-29, 1997. PMID: 9387021
- Gromada J, Holst J.J, Rorsman P. Cellular regulation of islet hormone secretion by the incretin hormone glucagon-like peptide 1. Pflugers Arch 435: 583-594, 1998. PMID: 9479010
- Habib A.M, Richards P, Cairns L.S, Rogers G.J, Bannon C.A, Parker H.E, Morley T.C, Yeo G.S, Reimann F, Gribble F.M. Overlap of Endocrine Hormone Expression in the Mouse Intestine Revealed by Transcriptional Profiling and flow Cytometry. Endocrinology 153: 3054-3065, 2012. PMID: 22685263
- Hansen L, Deacon C.F, Orskov C, Holst J.J. Glucagon-Like Peptide-1-(7-36) Amide Is Transformed to Glucagon-Like Peptide-1-(9-36) Amide by Dipeptidyl Peptidase IV in the Capillaries Supplying the L Cells of the Porcine Intestine. Endocrinology 140: 5356-5363, 1999. PMID: 10537167
- Hansen L, Hartmann B, Bisgaard T, Mineo H, Jorgensen P.N, Holst J.J. Somatostatin restrains the secretion of glucagon-like peptide-1 and -2 from isolated perfused porcine ileum. Am J Physio Endocrinol Metab 278:1010-1018, 2000. PMID: 10827002
- Hare K.J, Visboll T, Asmar M, Deacon C.F, Knop F.L, Holst J.J. The Glucagonostatic and Insulinotropic Effects of glucagon-Like Peptide 1 Contribute Equally to Its Glucose-Lowering Action. Diabetes 59: 1765-1770, 2010. PMID: 20150286
- Heller R.S, Kieffer T.J, Habener J.F. Insulinotropic glucagon-like peptide 1 receptor expression in glucagon-producing alpha-cells of the rat endocrine pancreas. Diabetes 46: 785-791, 1997. PMID: 9133545
- Heller R.S, Kieffer T.J, Habener J.F. Point mutations in the first and third intracellular loops of the glucagon-like peptide-1 receptor alter intracellular signaling. Biochem Biophys Res Commun 223: 624-632, 1996. PMID: 8687446
- Hirasawa A, Tsumaya K, Awaji T, Katsuma S, Adachi T, Yamada M, Sugimoto Y, Miyazaki S, Tsujimoto G. Free fatty acids regulate gut incretin glucagon-like peptide-1 secretion through GPR120. Nature Medicine 11: 90-94, 2005. PMID: 15619630
- Holst J.J, Hartmann C, Pedersen J. GLP 1/2, Enteroglucagon, Glicentin, and Oxyntomodulin. Handbook of Biologically Active Peptides 2nd Ed. 2013 pp 1241-1260 Ed by A.J. Kastin, Academic Press, Eisevier, 2013.
- Holst J.J. The Physiology of Glucagon-like Peptide 1. Physiol Rev 87: 1409-1439, 2007. PMID: 17928588
- Hörsch D, Göke R, Eissele R, Michel B, Göke B. Reciprocal cellular distribution of glucagon-like peptide-1 (GLP-1) immunoreactivity and GLP-1 receptor mRNA in pancreatic islets of rat. Pancreas, 14:290-294, 1997. PMID: 9094161
- Koehler J.A, Baggio L.L, Lamont B.J, Ali S, Drucker D.J. Glucagon-Like Peptide-1 Receptor Activation Modulates Pancreatitis-Associated Gene Expression But Does Not Modify the susceptibility to Experimental Pancreatitis in Mice. Diabetes 58: 2148-2161, 2009. PMID: 19509017
- Kreymann B, Williams G, Ghatei M.A, Bloom S.R. Glucagon-like Peptide-1 7-36: A Physiological Incretin in Man. Lancet 1300-1304, 1987. PMID: 2890903
- Kwan E.P, Gaisano H.Y. Glucagon-like Peptide 1 Regulates Sequential and Compound Exocytosis in Pancreatic Islet β-Cells. Diabetes 54: 27342743, 2005. PMID: 16123364
- Lamont B.J, Li Y, Kwan E, Brown T.J, Gaisano H, Drucker D.J. Pancreatic GLP-1 receptor activation is sufficient for incretin control of glucose metabolism in mice. J Clin Invest 122: 388-402, 2012. PMID: 22182839
- Larsson L, Holst J, Hakanson R, Sundler F. Distribution and Properties of glucagon Immunoreactivity in the Digestive Tract of Various Mammals: An Immunohistochemical and Immunochemical Study. Histochemistry 44: 281-290, 1975. PMID: 1102501
- Layer P, Holst J.J, Grandt D, Goebell H. Illeal release of glucagon-like peptide-1 (GLP-1). Association with inhibition of gastric acid secretion in humans. Dig Dis Sci 40: 1074-1082, 1995. PMID: 7729267
- Layer P, Peschel S, Schlesinger T, Goebell H. Human pancreatic secretion and intestinal motility: effects of ileal nutrient perfusion. Am J Physiol Gastrointest Liver Physiol 21: 196-201, 1990. PMID: 1689548
- Leech C.A, Dzhura I, Chepurny O.G, Kang G, Schwede F, Genieser H.G, Holz G.G. Molecular physiology of glucagon-like peptide-1 insulin secretagogue action in pancreatic β cells. Progress in Biophysics and Molecular Biology 107: 236-247, 2011. PMID: 21782840
- Malhotra R, Singh L, Eng J, Raufman J.P. Exendin-4, a new peptide from Heloderma suspectum venom, potentiates cholecystokinin-induced amylase release from rat pancreatic acini. Reg Peptides, 41: 149-156, 1992. PMID: 1279756
- Marguet D, Baggio L, Kobayashi T, Bernard A.M, Pierres M, Nielsen P.F, Ribel U, Wantanabe T, Drucker D.J, Wagtmann N. Enhanced insulin secretion and improved glucose tolerance in mice lacking CD26. PNAS 07: 6874-6879, 2000. PMID: 10823914
- Mathi S.K, Chan Y, Li X, Wheeler M.B. Scanning of the glucagon-like peptide-1 receptor localizes G protein-activating determinants primarily to the N terminus of the third intracellular loop. Mol Endocrinol 11: 424-432, 1997. PMID: 9092794
- Matsumura K, Miki T, Jhomori T, Gonoi T, Seino S. Possible role of PEPT1 in gastrointestinal hormone secretion. Biochem Biophys Res Commun 336: 1028-1032, 2005. PMID: 16181611
- Matveyenko A.V, Dry S, Cox H.I, Moshtaghian A, Gurlo T, Galasso R, Butler A.E, Butler P.C. Beneficial Endocrine but Adverse Exocrine Effects of Sitagliptin in the Human Islet Amyloid Polypeptide Transgenic Rat Model of Type 2 Diabetes. Diabetes 58: 1604-1615, 2009. PMID: 19403868
- McIntyre N, Holdsworth C.D, Turner D.S. New interpretation of oral glucose tolerance. Lancet 2: 20-21, 1964. PMID: 14149200
- Meier J.J, Nauck M.A, Kranz D, Holst J.J, Deacon C.F, Gaeckler D, Schmidt W.E, Gallwitz B. Secretion, degradation, and elimination of glucagon-like peptide 1 and gastric inhibitory polypeptide in patients with chronic renal insufficiency and healthy control subjects. Diabetes 53: 654-662, 2004. PMID: 14988249
- Miller L.J, Chen Q, Lam P, Pinon D.I, Sexton P.M, Abagyan R, Dong M. Refinement of Glucagon-like Peptide 1 Docking to Its Intact Receptor Using Mid-region Photolabile Probes and Molecular Modeling. J Biol Chem 286: 15895-15907, 2011. PMID: 21454562
- Mojsov S, Heinrich G, Wilson I.B, Ravazzola M, Orci L, Habener J.F. Preproglucagon Gene Expression in Pancreas and Intestine Diversifies at the Level of Post-translational Processing. J Biol Chem 261: 11880-11889, 1986. PMID: 3528148
- Mojsov S, Weir G.C, Habener J.F. Insulinotropin: Glucagon-like Peptide 1(7-37) Co-encoded in the Glucagon Gene Is a Potent Stimulator of Insulin Release in the Perfused Rat Pancreas. J Clin Invest, 79: 616-619, 1987. PMID: 3543057
- Montrose-Rafizadeh C, Avdonin P, Garant M.J, Rodgers B.D, Kole S, Yang H, Levine M.A, Schwindinger W, Bernier M. Pancreatic Glucagon-Like Peptide-1 Receptor Couples to Multiple G Proteins and Activates Mitogen-Activated Protein Kinase Pathways in Chinese Hamster Ovary Cells. Endocrinol 140: 1132-1140, 1999. PMID: 10067836
- Mortensen K, Christensen L.L, Holst J.J, Orskov C. GLP-1 and GIP are colocalized in a subset of endocrine cells in the small intestine. Regul Pept 114: 189-196, 2003. PMID: 12832109
- Nachnani J.S, Bulchandani D.G, Nookala A, Herndon B, Molteni A, Pandya P, Taylor R, Quinn T, Weide L, Alba L.M. Biochemical and histological effects of exendin-4 (exenatide) on the rat pancreas. Diabetologia 53: 153-159, 2010. PMID: 19756486
- Nadkarni P, Chepurny O.G, Holz G.G. Regulation of Glucose Homeostasis by GLP-1. Progress in Molecular Biology and Translational Science 21: 23-65, 2014. PMID: 24373234
- Nakamura T, Ito T, Uchida M, Hijioka M, Igarashi H, Oono T, Kato M, Nakamura K, Suzuki K, Jensen R.T, Takayanagi R. PSCs and GLP-1R: occurrence in normal pancreas, acute/chronic pancreatitis and effect of their activation by a GLP-1R agonist. Laboratory Investigation 94: 63-78, 2013.
- Nauck M.A, Freidrich N. Do GLP-1-Based Therapies Increase Cancer Risk? Diabetes Care 36: S245-S252, 2013. PMID: 23882053
- Nauck M.A, Homberger E, Siegel E.G, Allen R.C, Eaton R.P, Ebert R, Creutzfeldt W. Incretin Effects of Increasing Glucose Loads in Man Calculated from Venous Insulin and C-Peptide Reponses. J of Clin Endocrinology and Metabolism 63: 492- 498, 1986. PMID: 3522621
- Nauck M.A, Niedereicholz U, Ettler R, Holst J.J, Orskov C, Ritzel R, Schmiegel W.H. Glucagon-like peptide 1 inhibition of gastric emptying outweighs its insulinotropic effects in healthy humans. Am J Physiol 273: E981-E988, 1997. PMID: 9374685
- Nilsson O, Bilchik A.J, Goldenring J.R, Ballantyne G.H, Adrian T.E, Modlin I.M. Distribution and immunocytochemical colocalization of peptide YY and enteroglucagon in endocrine cells of the rabbit colon. Endocrinology 129: 139-148, 1991. PMID: 1675986
- Nyborg N.C, Molck A.M, Madsen L.W, Knudsen L.B. The Human GLP-1 Analog Liraglutide and the Pancreas Evidence for the Absence of Structural Pancreatic Changes in Three Species. Diabetes 61: 1243-1249, 2012. PMID: 22338093
- Orskov C, Bersani M, Johensen A.H, Hejrup P, Holst JJ. Complete Sequences of Glucagon-like Peptide-1 from Human and Pig Small Intestine. J Biol Chem, 22: 12826-12829, 1989. PMID: 2753890
- Orskov C, Poulsen S.S. Glucagonlike peptide-1-(7-36)-amide receptors only in islets of Langerhans. Autoradiographic survey of extracerebral tissues in rats. Diabetes 40: 1292-1296, 1991. PMID: 1657666
- Orskov C, Rabenhoj L, Wettergren A, Kofod H, Holst J.J. Tissue and plasma concentration of amidated and glycine-extended glucagon-like peptide 1 in humans. Diabetes 43: 535-539, 1994. PMID: 8138058
- Orskov C, Wettergren A, Holst J.J. Secretion of the incretin hormones glucagon-like peptide-1 and gastric inhibitory polypeptide correlates with insulin secretion in normal man throughout the day. Scand J Gastroenterol 31: 665, 670, 1996. PMID: 8819215
- Parker H.E, Adriaenssens A, Rogers G, Richards P, Koepsell H, Reimann F, Gribble F.M. Predominant role of active versus facilitative glucose transport for glucagon-like peptide-1 secretion. Diabetologia 55: 2445-2455, 2012. PMID: 22638549
- Pyke C, Knudsen LB. The Glucagon-Like Peptide-1 Receptor—or Not? Endocrinology 154: 4-8, 2013. PMID: 23267050
- Raufman J.P, Singh L, Eng J. Exendin-3, a novel peptide from Heloderma horridum venom, interacts with vasoactive intestinal peptide receptors and a newly described receptor on dispersed acini from guinea pig pancreas. Description of exendin-3(9-39) amide, a specific exendin receptor antagonist. J Biol Chem 15: 2897-2902, 1991. PMID: 1704369
- Raufman J.P, Singh L, Singh G, Eng J. Truncated Glucagon-like Peptide-1 Interacts with Exendin Receptors on Dispersed Acini from Guinea Pig Pancreas. J Biol Chem 267: 21432-21437, 1992. PMID: 1328231
- Reimann F, Habib A.M, Tolhurst G, Parker H.E, Rogers G.J, Gribble F.M. Glucose Sensing in L Cells: A Primary Cell Study. Cell Metabolism 8: 532-539, 2008. PMID:19041768
- Rocca A.S, Brubaker P.L. Role of the vagus nerve in mediating proximal nutrient-induced glucagon-like peptide-1 secretion. Endocrinology 140: 1687-1694, 1999. PMID:10098504
- Rouille Y, Martin S, Steiner D.F. Differential processing of proglucagon by the subtillsin-like prohormone convertases PC2 and PC3 to generate either glucagon or glucagon-like peptide. J Biol Chem 270: 26488-26496, 1995. PMID: 7592866
- Ryder R.E. The potential risks of pancreatitis and pancreatic cancer with GLP-1 based therapies are far outweighed by the proven and potential (cardiovascular) benefits. Diabet Med 30: 1148-115, 2013. PMID: 24073725
- Scheen A. Gliptins (dipeptidyl peptidase-4 inhibitors) and risk of acute pancreatitis. J Expert Opin Drug Saf, 12: 545-557, 2013. PMID: 23621381
- Scirica B.M, Bhatt D.L, Braunwald E, Steg P.G, Davidson J, Hirshberg B, Ohman P, Frederich R, Wiviott S.D, Hoffman E.B, Cavender M.A, Udell J.A, Desai N.R, Mosenzon O, McGuire D.K, Ray K.K, Leiter L.A, Raz I, SAVOR-TIMI 53 Streering Committee and Investigators. Saxagliptin and cardiovascular outcomes in patients with type 2 diabtes mellitus. N Engl J Med 369: 1317-1326, 2013. PMID: 23992601
- Scrocchi L.A, Brown T.J, MaClusky N, Brubaker P.L, Auerbach A.B, Joyner A.L, Drucker D.J. Glucose intolerance but normal satiety in mice with a null mutation in the glucagon-like peptide 1 receptor gene. Nat Med. 2: 1254-1258,1996. PMID: 8898756
- Sheikh A. Direct cardiovascular effects of glucagon like peptide-1. Diabetology & Metabolic Syndrome 47, 2013. PMID: 23988189
- Singh G, Eng J, Raufman J.P. Use of 125I-[Y39]exendin-4 to characterize exendin receptors on dispersed pancreatic acini and gastric chief cells from guinea pig. Regulatory Peptides 53: 47-59, 1994. PMID: 7800858
- Song W.J, Seshadri M, Ashraf U, Mdluli T, Mondal P, Keil M, Azevedo M, Kirschner L.S, Stratakis C.A, Hussain M.A. Snapin Mediates Incretin action and Augments Glucose-Dependent Insulin Secretion. Cell Metabolism 13: 308-319, 2011. PMID: 21356520
- Stoffers D.A, Kieffer T.J, Hussain M.A, Drucker D.J, Bonner-Weir S, Habener J.F, Egan J.M. Insulinotropic Glucagon-Like Peptide 1 Agonists Stimulate Expression of Homeodomain Protein IDX-1 and Increase Islet Size in Mouse Pancreas. Diabetes 49: 741-748, 2000. PMID: 10905482
- Tatarkiewicz K, Belanger P, Gu G, Parkes D, Roy D. No evidence of drug-induced pancreatitis in rats treated with exenatide for 13 weeks. Diabetes Obesity Metabolism 15: 417-426, 2012. PMID: 23163898
- Tatarkiewicz K, Smith P.A, Sablan E.J, Polizzi C.J, Aumann D.E, Villescaz C, Hargrove D.M, Gedulin B.R, Lu M.G, Adams L, Whisenant T, Roy D, Parkes D.G. Exenatide does not evoke pancreatitis and attenuates chemically induced pancreatitis in normal and diabetic rodents. Am J Physiol Endocrinol Metab 299: 1076-1086, 2010. PMID: 20923958
- Thorens B. Expression cloning of the pancreatic β cell receptor for the gluco-incretin hormone glucagon-like peptide. Proc Natl Acad Sci USA 89: 8641-8645, 1992. PMID: 1326760
- Thornton K, Gorenstein D.G. Structure of glucagon-like peptide (7-36) amide in a dodecylphosphocholine micelle as determined by 2D NMR. Biochemistry, 33:3532-3539, 1994. PMID: 8142350
- Tolhurst G, Heffron H, Lam Y.S, Parker H.E, Habib A.M, Diakogiannaki E, Cameron J, Grosse J, Reimann F, Gribble F.M. Short-Chain Fatty Acids Stimulate Glucagon-Like Peptide-1 Secretion via the G-Protein-Coupled Receptor FFAR2. Diabetes 61: 364-371, 2012. PMID: 22190648
- Tolhurst G, Zheng Y, Parker H.E, Habib A.M, Reimann F, Gribble F.M. Glutamine triggers and potentiates glucagon-like peptide-1 secretion by raising cytosolic Ca2+ and cAMP. Endocrinology 152L 405-413, 2011. PMID: 21209017
- Tornehave D, Kristensen P, Rømer J, Knudsen L.B, Heller R.S. Expression of the GLP-1 receptor in mouse, rat, and human pancreas. J Histochem Cytochem 56:841-851, 2008. PMID: 18541709
- Tschen S.I, Georgia S, Dhawan S, Bhushan A. Skp2 Is Required for Incretin Hormone-Mediated β-Cell Proliferation. Molecular Endocrinology 25: 2134-2143, 2011. PMID: 21980072
- Turton M.D, O’Shea D, Gunn L, Beak S.A, Edwards C.M, Meeran K, Choi S.J, Taylor G.M, Heath M.M, Lambert P.D, Wilding J.P, Smith D.M, Ghatel M.A, Herbert J, Bloom S.R. A role for glucagon-like peptide-1 in the central regulation of feeding. Nature 379: 69-72, 1996. PMID: 8538742
- Vilsboll T, Agerso H, Krarup T, Holst J.J. Similar elimination rates of glucagon-like peptide-1 in obese type 2 diabetic patients and healthy subjects. J Clin Endocrinol Metab 88: 220-224, 2003. PMID: 12519856
- Vrang N, Jelsing J, Simonsen L, Jensen A.E, Thorup I, Soeborg H, Knudsen L.B. The effects of 13 wk of liraglutide treatment on endocrine and exocrine pancreas in male and female ZDF rats: a quantitative and qualitative analysis revealing no evidence of drug-induced pancreatitis. Am J Physiol Endocrinol Metab 303: 253-264, 2012. PMID: 22589391
- Waget A, Cabou C, Masseboeuf M, Cattan P, Armanet M, Karaca M, Castel J, Garret C, Payros G, Maida A, Sulpice T, Holst J.J, Drucker D.J, Magnan C, Burcelin R. Physiological and Pharmacological Mechanisms through which the DPP-4 Inhibitor Sitagliptin Regulates Glycemia in Mice. Endocrinology 152: 3018-3029, 2011. PMID: 21673098
- Wan S, Coleman F.H, Travagli R.A. Glucagon-like peptide-1 excites pancreas-projecting preganglionic vagal motoneurons. Am J Physiol 292: G1474-G1482, 2007. PMID:17322063
- Weber V, Fehmann H, Goke R, Goke B. Effect of Proglucagon-Derived Peptides on amylase Release from Rat Pancreatic Acini. Int J Pancreatology 7: 325-330, 1990.
- Weir G.C, Mojsov S, Hendrick G.K, Habener J.F. Glucagonlike Peptide 1 (7-37) Action on Endocrine Pancreas. Diabetes 38: 338-342, 1989. PMID: 2645190
- Wettergren A, Schjoldager B, Mortensen P.E, Myhre J, Christiansen J, Holst J.J. Truncated GLP-1 (Proglucagon 78-107-Amide) Inhibits Gastric and Pancreatic Functions in Man. Digestive Diseases and Sci 38: 665-673, 1993. PMID: 8462365
- Wettergren A, Wojdemann M, Holst J.J. Glucagon-like peptide-1 inhibits gastropancreatic function by inhibiting central parasympathetic outflow. Am J Physiol Gastrointest Liver Physiol 38: 6984-6992, 1998. PMID: 9815028
- Wheeler M.B, Lu M, Dillon J.S, Leng X.H, Chen C, Boyd III A.E. Functional Expression of the Rat Glucagon-Like Peptide-1 Receptor, Evidence for Coupling to both Adenylyl Cyclase and Phospholipase-C. J Endocrinol, 133: 57- 62, 1993. PMID: 8391428
- Winzell M.S, Ahren B. Durable islet effects on insulin secretion and protein kinase A expression following exendin-4 treatment of high-fat diet-fed mice. J Mol Endocrinol 40: 93-100, 2008. PMID:18234911
- Wu T, Rayner C.K, Jones K, Horowitz M. Dietary Effects on Incretin Hormone Secretion. Vitamins and Hormones 84: 81-110, 2010. PMID: 21094897
- Xu G, Kaneto H, Lopez-Avalos M.D, Weir G.C, Bonner-Weir S. GLP-1/exendin-4 facilitates beta-cell neogenesis in rat and human pancreatic ducts. Diabetes Res Clin Pract 73:107-110, 2006. PMID: 16406191
- Yu X, Tang H, Huang L, Yang Y, Tian B, Yu C. Exenatide-Induced Chronic Damage of Pancreatic Tissue in Rats. Pancreas 41: 1235-1240, 2012. PMID: 22836857
- Yusta B, Baggio L.L, Estall J.L, Koehlet J.A, Holland D.P, Li H, Pipeleers D, Ling Z, Drucker D.J. GLP-1 receptor activation improves β cell function and survival following induction of endoplasmic reticulum stress. Cell Metabolism 4: 391-406, 2006. PMID:17084712
- Zhou J, Montrose-Rafizadeh C, Janczewski A.M, Pineyro M.A, Sollott S.J, Wang Y, Egan J.M. Glucagon-Like Peptide-1 Does not Mediate Amylase Release From AR42J Cells. J Cell Physiol 181: 470-478, 1999. PMID:10528233