Methods Type:
Entry Version:
Citation:
Pancreapedia: Exocrine Pancreas Knowledge Base, DOI: 10.3998/panc.2013.2
Attachment | Size |
---|---|
![]() | 284.44 KB |
I. Introduction
Acute pancreatitis is one of the most common diseases in gastroenterology. The incidence of acute pancreatitis per 100,000 population ranges from 10 to 46 per year. Two percent of all patients admitted to hospital are diagnosed with acute pancreatitis. Nevertheless even in the 21st century neither the etiology nor the pathophysiology of the disease are fully understood and causal treatment options are not available. This fact has prompted numerous researchers to study the initial triggering events of acute pancreatitis in order to develop new treatment strategies. Much of our current knowledge regarding the onset of pancreatitis was not gained from studies involving the human pancreas or patients with pancreatitis but from animal or isolated cell models. There are several reasons why these models have been used: 1) the pancreas is a rather inaccessible organ because of its anatomical location in the retroperitoneal space. Unlike the colon or stomach, biopsies of human pancreas are difficult to obtain for ethical and medical reasons. 2) Patients who are admitted to hospital with acute pancreatitis have usually passed through the initial stages of the disease where the triggering early events could have been studied. Particularly the autodigestive process that characterizes this disease has remained a significant impediment for investigations that address initiating pathophysiological events. Therefore, the pathophysiological trigger mechanisms have mostly been studied in animal models of the disease, before randomized placebo controlled trials to evaluate new therapeutic concepts in humans could be initiated. Experimental models are now irreplaceable tools in studying etiological factors, pathophysiology, new diagnostic tools as well as treatment options in acute pancreatitis. The advantages of animal models are the possibility to isolate specific aspects of a complex and varying disease course, the high degree of standardization and reproducibility, and the reduction of the required sample size eliminating variability in the study population.
Animal models can be grouped into invasive in vivo models, non invasive in vivo models and ex vivo models. The mode of onset and development, the disease severity, the extent of inflammation and the associated mortality vary considerably among different models. Here we focus on the model of secretagogue induced pancreatitis.
Secretagogue induced pancreatitis
It is generally believed that the morphologic changes that characterize acute pancreatitis result from digestion of the gland by enzymes that are normally synthesized and secreted by pancreatic acinar cells [1, 2, 3]. Evidence which supports this notion includes the observation that a) the morphological changes of severe pancreatitis resemble those that are typical of digestive necrosis [4]; b) pancreatic acinar cells synthesize digestive enzymes, which, when activated, lead to digestive necrosis of the gland [5]; and c) activated digestive enzymes have been detected within the gland during severe pancreatitis [6]. Most of the potentially harmful digestive proteases of acinar cells are normally synthesized and secreted as inactive zymogens and activated physiologically in the duodenum, a process initiated by brushborder enzymes [7].
As early as in 1895 Mouret et al. reported that excessive cholinergic stimulation is associated with the development of pancreatic injury determined by acinar cell vacuolization and necrosis [8]. Mouret suggested that the activation of trypsin might be actively involved in the development of acute pancreatitis. This hypothesis was in accordance with the work of Hans Chiari who in 1896 proposed that autodigestion as the consequence of premature zymogen activation was the pathological mechanism underlying acute pancreatitis [1]. Subsequently experimental animal models employing cholinergic agonists like carbamylcholine (carbachol), CCK and its analogues as well as scorpion venom were shown to induce pancreatic injury in a time- and dose-dependent manner [9, 10, 11, 12, 13]. In rodents CCK plays a major role in regulating exocrine pancreatic secretion after stimulation by food ingestion. However, human pancreatic acinar cells respond only poorly to direct CCK stimulation but are mostly regulated by cholinergic pathways that involve neurogenic CCK stimulation [14]. Lampel and Kern characterised in 1977 the clinical and biochemical pattern of acute interstitial pancreatitis in rats after administration of excessive doses of pancreatic secretagogue and thus established the model of secretagogue-induced pancreatitis [15]. The most prominent clinical characteristic is the development of excessive edema as early as one hour after the onset of the disease [16]. Since that time the model of caerulein (a CCK analogue derived from the Australian tree frog Litoria caerulea, formerly Hyla caerulea) -induced pancreatitis in rodents has been widely used and is one of the best characterized models.
The primary physiological effect of CCK and its analogues on the pancreas is to stimulate protein rich secretion and it has a lesser effect on fluid and electrolyte secretion. Doses of CCK that lead to continued maximal stimulation of enzyme secretion are associated with increased rates of protein synthesis and the movement of newly synthesized proteins through the secretory pathway. The increase in protein synthesis is outpaced by the rate of protein secretion. Thus, following stimulation with maximal secretory doses of caerulein, the enzyme stores of the exocrine pancreas may be reduced by 75% within several hours. Increasing the concentration of CCK by an order of magnitude over the levels that produce maximal secretion is known as supraoptimal stimulation, supramaximal stimulation or hyperstimulation [16]. Compared to maximal stimulation, supramaximal stimulation generates a distinct pancreatic response that includes diminished secretion, accumulation of secretory proteins within the pancreas and pancreatic injury. The route of administration for caerulein that induces acute pancreatitis differs in various rodents as does the severity of the disease [17, 18, 19, 20]. While in rats caerulein can be continuously, intravenously infused either via a polyethylene catheter placed into the external jugular vein or into the tail vein, in mice caerulein is generally injected repeatedly into the peritoneal cavity [21]. The caerulein concentration that results in pancreatic edema, increased serum levels of pancreatic enzymes, inflammation and necrosis ranges between 5 to 10 µg/kg/h in rats and thereby exceeds the maximal secretory doses by 10 to 20 fold. Maximal pancreatic injury occurs after 12 h of continuous infusion but changes can be monitored already 15 minutes after the start of the caerulein infusion and resolve spontaneously after 24 to 48 h. One of the earliest consequences of hyperstimulation is the formation of pancreatic edema. This increase in pancreatic fluid, which occurs within the first hour of caerulein hyperstimulation, is probably the result of several factors: increased vascular permeability [22,23,24], increased hydrostatic pressure from the constriction of small vessels and increased tissue oncotic pressure from the interstitial release of pancreatic enzymes and hydrolytic products. At present, the exact mechanism which leads to the formation of massive edema is not fully understood, but from its time-course a primary signalling effect of caerulein, which does not parallel acinar cell damage, is suggested. Under the conditions of supramaximal caerulein stimulation, secretion of zymogens into the pancreatic duct is virtually abolished; premature zymogen activation can be observed after a sustained intracellular calcium rise and a breakdown of the actin cytoskeleton [25]. These events lead to a systemic inflammatory response syndrome which includes extrapancreatic damage such as pancreatitis related lung injury [26].
The caerulein model of experimental pancreatitis is now widely used for the analysis of intracellular events in the early phase of pancreatitis. The model offers several advantages: it is non-invasive since no surgical intervention affecting the bile duct or pancreatic duct is necessary, it allows easily controlled grades of injury, it is highly reproducible and it is applicable in several animals species such as mice, rats, hamsters and dogs. The most important limitation of this model is that only a mild, self limited disorder is generated, which limits its use to study the destructive effects of the disease which confer clinical morbidity and mortality [27- 33]. (Figure 1).
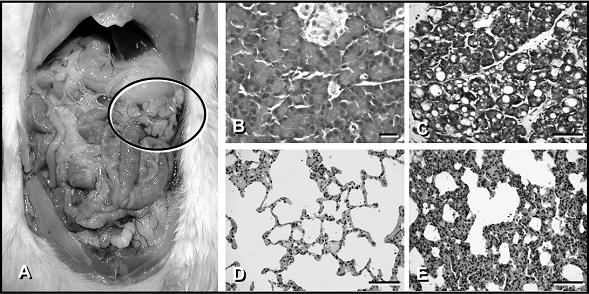
Figure 1. The left panel illustrates the macroscopic findings of the pancreas after supramaximal caerulein stimulation in rats. Note the gelatinous mass of the pancreas marked with a black circle, which comprised 90% water content. Panel B and C Light microscopy of pancreatic tissue: Pancreatic tissue was fixed, embedded in paraffin and sections were stained with hematoxylin and eosin. Note the development of interstitial edema, the infiltration of inflammatory cells and the development of vacuoles in the animals treated with supramaximal concentrations of caerulein [Panel C]. Panel B: Tissue section from control animals. Panel D and E: Light microscopy of lung tissue. Lung tissue was fixed, embedded and stained similar to pancreas. The morphological changes in the lungs of animals with caerulein-induced pancreatitis [Panel E] consisted of alveolar fluid accumulation and a progressive thickening, hyperemia and neutrophil infiltration of the interalveolar tissue. Panel D: Lung tissue form a control animal.
II. Materials
- Rodents of any kind:
All rodents (mice, rat) independent of the gender develop pancreatitis upon supramaximal secretagogue stimulation. However, for mice Halangk and co-workers (unpublished data) could show that the extent of pancreatic injury as well as systemic injury differs between in- and outbred mice strains. (Note 1) This can also be observed for the time course of pancreatitis. For rats animals with weight between 200 to 300 g and an approximate age of 12 to 36 weeks should be used. The weight of the mice used for an experiment should usually lie between 20 to 25g and the recommended age is 12 to 36 weeks. For treatment and control groups always the same strain, age and sex of animals should be used to allow for proper comparisons. (Note 2) Animals should allowed to adapt to their experimental environment for at least 3 days as transport stress can render the animals unresponsive to caerulein and they may not develop pancreatitis. - Anesthesia:
Ketamin Ketanest, Merck Serono
Xylazine Rompun 2%, Bayer Health Care
Ether Sigma, Taufkirchen
Pentobarbital Nembutal, Bayer Health Care - Syringes (Perfusor-Syringe OPS 50 ml, Fa. Braun Melsungen AG, D-3508 Melsungen)
- Jugular vein catheter: Portex 30m, non-sterile Polythene tubing, Ref.: 800/110/200, Diameter 58 µm.
- Surgical suture material: Ethicon Mersilene Polyester, 3 Metric 2-0, Ethicon Prolene monofil, 1.5 Metric 4-0, 45 cm, Ethicon, D-2000 Norderstedt.
- Amylase/Lipase Kits: P-AMYL from Roche/Hitachi Ref.: 11555812316 and LIP Roche/Hitachi Ref.: 11821792216 from Roche Diagnostics Mannheim or Indianapolis
- Trypsin substrate: R110 (CBZ-Ile-Pro-Arg) from initrogen Cat# R6505
III. Reagents:
- Caerulein Sigma, Taufkirchen, Germany
- PBS: 13.7 mM NaCl,
2.7 mM KCl,
80.9 mM Na2HPO4,
1.5 mM KH2PO4, pH 7.4 (HCl)
Caerulein can be dissolved in PBS or 0.9% NaCl in a concentration of 100µg/ml. This stock solution can be stored at -20°C. Before starting the experiments, the stock solution is diluted in PBS or 0.9% NaCl to a final working concentration which depends on the animal weight and experimental conditions.
IV. Methods:
Mice:
After delivery mice weighing 20 to 25 gr should be allowed to adjust to the local conditions of the animal facility for 5 to 7 days. Before the experiment mice should be starved for 12 hours and allowed water ad libitum. (Note 3) Animals are injected intraperitoneally into the right lower quadrant with a 50µg/kg/bw of caerulein dissolved in either PBS or 0.9% saline in a volume of 100 µl. Injections take place in hourly intervals up to 7 injections, but in some cases the protocol differs in times of injections up to 12 h, or over a time period of two days. Severity of pancreatitis depends on the number of injections and a wide range has been published. For the period of injections, it is not needed to give the mice an anesthesia or pain medication. The level of pain is quite moderate in the model of Caerulein induced pancreatitis compared to other models like Tauchocholate-induced pancreatitis. However, different countries may require pain medication if pancreatitis is induced. In this case we suggest to use metamizol added to the drinking water (200mg/kg bodyweight dissolved in 3ml water/d which resembles the usually fluid intake per day). Animals are sacrificed at the respected time course. Maximal changes consistent with pancreatitis can be expected 8 hrs after the first injection of caerulein. Males as well as female mice can be used for the experiments. However using only one sex allows for better standardization of results.
Analgo-sedation mice:
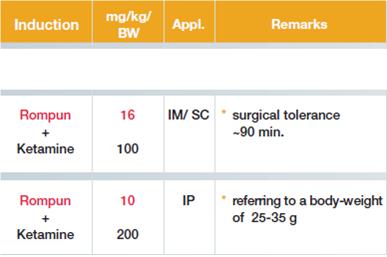
Rats:
Rats weighing 200 to 300 g are analgo-sedated with ketamine and xylazine. A midline incision at the neck of the rat with a scalpel is performed, followed by blunt preparation of the extra-jugular vein next to the carotic artery. The jugular vein is then ligated in the cranial part to prevent bleeding using Ethicon Mersilene Polyester, 3 Metric 2-0 and a second suture is prepared but not closed in the caudal part of the jugular vein close to the thoracic aperture. (Figure 2). A small incision to the jugular vein is done and a Portex catheter with a diameter of 58 µm is put into the vein. The catheter is fixed using the suture material described above on the sternocleidomastoideal muscle and the skin is closed using Ethicon Prolene monofil, 1.5 Metric 4-0, 45 cm. Caerulein dissolved in PBS or saline in a concentration of 10 µg/kg bw/h is infused via an infusion pump in a volume of 1ml per h. Animals are sacrificed by lethal bleeding under analog-sedation. An alternative method to induce Caerulein pancreatitis in rats is similar to the mouse model, hourly i.p. injections of Caerulein (50µg/kg/bw). The advantage here is that anesthesia as well as pain medication over the period of injections is not needed.
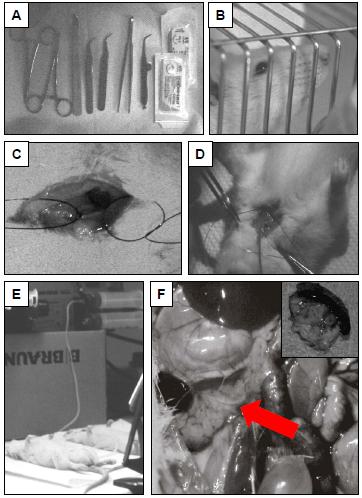
Figure 2. Induction of caerulein induced pancreatitis in rats: A: necessary instruments from left to right: acutenaculum (two needle holders), scalpel, two sharp forceps for blunt preparation of the extra-jugular vein, one blunt forceps for closing the skin, small scissor for the incision of the extra-jugular vein. B: male Wistar rat in cage, C: dissected and prepared extra-jugular vein D: cranial ligation of the jugular vein and fixing of the portex polyethylen catheter. E: constant infusion of supramaximal caerulein with an infusion volume of 1 ml per hour, F: rat open abdomen after two hours of infusion of caerulein. Red arrow: pancreas with edema, Insert: harvested pancreas.
Analgo-sedation:
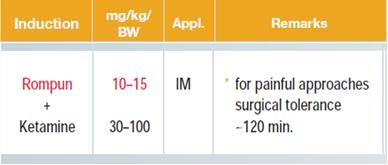
Markers for pancreatitis
To evaluate the severity of pancreatitis, serum activity of lipase and amylase can be measured by a colorimetric assay. Serum lipase activity as well as amylase activity is measured as a kinetic reation over 30 min by use of a photometric plate reader. For both assays kits are available from Roche Hitachi. After induction of pancreatitis, amylase as well as lipase is slightly increased after 1 h up to a maximum increase after 8 h over 3 fold increase of amylase activity and over 5-8 fold increase of lipase activity compared to untreated control animals. An increase of intrapancreatic trypsin activity can be measured by fluorogenic substrates. We use a Rhodamine 110 based bis-peptide substrate (CBZ-Ile-Pro-Arg), in a kinetic assay over 1 h. Pancreatic homogenates were prepared in 100 mM Tris, 5 mM CaCl2, pH 8 without any protease inhibitors. It is necessary to work fast and on ice to prevent additional protease activation. The measurement of trypsin activity is a fluorometric assay with excitation at 498 nm and a peak emission at 521 nm. The activity of pancreatic trypsin increases during pancreatitis to about 5 fold increase after 8 h compared to the untreated controls.
V. Notes
- C57BL6 mice (Black 6) give the highest response with regard to protease activity.
- Unstarved animals do get less severe pancreatitis as zymogen stores may be emptied of proteases after feeding.
- While caerulein induced pancreatitis in rats is characterized by large vacuoles, mild local pancreatic damage and a mild systemic inflammatory response, lethality can be achieved in mice if repetitive caerulein application is repeated daily over the time period of one week.
References
- Chiari H: Über die Selbstverdauung des menschlichen Pankreas. Z Heilk 17, 69-96, 1896.
- Gorelick FS, Adler G, Kern HF: Cerulein-induced pancreatitis. In: The Pancreas: Biology, Pathobiology, and Disease, edited by V. L. W. Go, E. P. DiMagno, J. D. Gardner, E. Lebenthal, H. A. Reber, and G. A. Scheele. New York: Raven Press, Ltd., 1993, p. 501-526.
- Lerch MM, Saluja AK, Runzi M, Dawra R, Saluja M, Steer ML. Acute necrotising pancreatitis in the opossum: earliest morphologic changes involve acinar cells. Gastroenterology 103: 205-213, 1992. PMID: 1612327
- Foulis AK: Histological evidence of initiating factors in acute necrotizing pancreatitis in man. J Clin Path 33:1125-1131, 1980. PMID: 7451660
- Bialek R, Willemer S, Arnold R, Adler G. Evidence of intracellular activation of serine proteases in acute cerulein-induced pancreatitis in rats. Scand J Gastroenterol 26: 190-196, 1991. PMID: 1707179
- Luthen R, Niederau C, Grendell JH. Intrapancreatic zymogen activation and levels of ATP and glutathione during caerulein pancreatitis in rats. Am J Physiol 268: G592-G604, 1995. PMID: 7537455
- Rinderknecht H: Activation of pancreatic zymogens. Normal activation, premature intrapancreatic activation, protective mechanisms against inappropriate activation. Dig Dis Sci 31: 314-21, 1986. PMID: 2936587
- Mouret J. Contribution á l’etude des cellules glandulaires (pancreas) J Anat Physiol 1895 ; 31: 221-236.
- Adler G, Gerhards G, Schick J, Rohr G, Kern HF. Effects of in vivo cholinergic stimulation of rat exocrine pancreas. Am J Physiol 1983; 244: G623-629. PMID: 6190409
- Niederau C, Ferrell LD, Grendell JH. Caerulein-induced acute necrotizong pancreatitis in mice: protective effects of proglumide, benzotript, and secretin. Gastroenterology 1985; 88: 1192-1204. PMID: 2984080
- Saluja A, Saito I, Saluja M, Houlihan MJ, Powers RE, Meldolesi J, Steer M. In vivo rat pancreatic acinar cell function during supramaximal stimulation with caerulein. Am J Physiol 1985; 249: G702-G710. PMID: 2417493
- Watanabe O, Baccino FM, Steer ML, Meldolesi J. Supramaximal caerulein stimulation and ultrastructure of rat pancreatic acinar cell: early morphological changes during development of experimental pancreatitis. Am J Physiol 1984; 246: G457-467. PMID: 6720895
- Pantoja JL, Renner IG, Abramson SB, Edmondson HA, Production of acute hemorrhagic pancreatitis in the dog using venom of the scorpion, Buthus quinquestriatus. Dig Dis Sci 1983; 28: 429-439. PMID: 6839906
- Ji B, Bi Y, Simenone D, Mortensen RM, Logsdon CD. Human pancreatic acinar cells do not respond to cholecystokinin. Pharmacology and toxicology 2002: 91: 327-332. PMID: 12688376
- Lampel M, Kern HF. Acute interstitial pancreatitis in the rat induced by excessive doses of a pancreatic secretagogue. Virchows Arch path Anat Histol 1977; 373: 97-117. PMID: 139754
- Scheele G, Palade G. Studies on the pancreas of the guinea pig – parallel discharge of exocrine enzyme activities. J Biol Chem 1975; 250: 2660-2670. PMID: 1123325
- Lerch MM, Albrecht E, Ruthenburger M, Mayerle J, Halangk W, Kruger B. Pathophysiology of alcohol-induced pancreatitis. Pancreas. 2003 Nov; 27(4):291-6. PMID: 14576489
- Lerch MM, Lutz MP, Weidenbach H, Muller-Pillasch F, Gress TM, Leser J, Adler G. Dissociation and reassembly of adherens junctions during experimental acute pancreatitis. Gastroenterology. 1997 Oct; 113(4):1355-66. PMID: 9322531
- Lerch MM, Saluja AK, Runzi M, Dawra R, Steer ML. Luminal endocytosis and intracellular targeting by acinar cells during early biliary pancreatitis in the opossum. J Clin Invest. 1995 May; 95(5):2222-31. PMID: 7537759
- Lerch MM, Saluja AK, Dawra R, Saluja M, Steer ML. The effect of chloroquine administration on two experimental models of acute pancreatitis. Gastroenterology. 1993 Jun; 104(6):1768-79. PMID: 8500736
- Adler G, Rohr G, Kern HF. Alteration of membrane fusion as a cause of acute pancreatitis in the rat. Dig Dis Sci 1982; 27: 993-1002. PMID: 7140496
- Lerch MM, Weidenbach H, Gress TM, Adler G. Effect of kinin inhibition in experimental acute pancreatitis. Am J Physiol. 1995 Oct; 269(4 Pt 1):G490-9. PMID: 7485500
- Weidenbach H, Lerch MM, Gress TM, Pfaff D, Turi S, Adler G. Vasoactive mediators and the progression from oedematous to necrotising experimental acute pancreatitis. Gut. 1995 Sep; 37(3):434-40. PMID: 7590444
- Jungermann J, Lerch MM, Weidenbach H, Lutz MP, Kruger B, Adler G. Disassembly of rat pancreatic acinar cell cytoskeleton during supramaximal secretagogue stimulation. Am J Physiol. 1995; 268: G328-38. PMID: 7864130
- Halangk W, Lerch MM, Brandt-Nedelev B, et al: Role of cathepsin B in intracellular trypsinogen activation and the onset of acute pancreatitis. J Clin Invest 106:773-781, 2000. PMID: 10995788
- Lerch MM, Adler G: Experimental pancreatitis. Curr Opinion Gastroenterology 9: 752-759, 1993.
- Schnekenburger J, Mayerle J, Krüger B, Buchwalow I, Weiss FU, Albrecht E, Samoilova VE, Domschke W, Lerch MM. Protein tyrosine phosphatase kappa and SHP-1 are involved in the regulation of cell-cell contacts at adherens junctions in the exocrine pancreas. Gut. 2005 Oct; 54(10):1445-55. PMID: 15987791
- Lerch MM, Adler G: Experimental animal models of acute pancreatitis. Int J Pancreatol 15: 159-170, 1994. PMID: 7930776
- M.M. Lerch, W. Halangk, B. Krüger. The role of cysteine proteases in intracellular pancreatic serine protease activation. Adv Exp Med Biol 2000; 477:403-411. PMID: 10849766
- F. Mooren, S. Turi, D. Günzel, W.R. Schlue, W. Domschke, J. Singh, M.M. Lerch. Calcium-magnesium interactions in pancreatic acinar cells. FASEB Journal 2001; 15:660-672. PMID: 11259384
- B. Krüger, I.A. Weber, E. Albrecht, F.C. Mooren, M.M. Lerch. Effect of hyperthermia on premature intracellular trypsinogen activation in the exocrine pancreas. Biochem. Biophys. Res. Comm. 2001; 282:159-165. PMID: 11263986
- W. Halangk, B. Krüger, M. Ruthenbürger, J. Stürzebecher, H. Lippert, M.M. Lerch. The role of trypsin in premature, intrapancreatic trypsinogen activation and inactivation of trypsin activity. Am. J. Physiol. Gastrointest. Liver Physiol. 2002; 282:G367-74. PMID: 11804859