Methods Type:
Entry Version:
Citation:
Pancreapedia: Exocrine Pancreas Knowledge Base, DOI: 10.3998/panc.2011.5
Attachment | Size |
---|---|
![]() | 349.17 KB |
Use of cell permeabilization methodology including cracked cells, cell sheets, detergents (saponin) and the cholesterol-binding b-hemolytic cytolysins a-toxin, streptolysin O (SLO) and perfringolysin O (PFO) has proven to be an invaluable tool in identifying and characterizing the role of soluble regulatory proteins and membrane- impermeant chemicals that modulate cellular function. Cell permeabilization has been used in numerous secretory cell types including neural, endocrine, immune, and exocrine cells (1,4-6,10-15,17-29,34-41). Permeabilized preparations provide the distinct advantage of allowing the acute introduction of small molecules and proteins into the cytoplasm. Clamping the Ca2+ concentration at a desired level is used to stimulate secretion, as only those cells that are permeabilized will respond to the ion. Moreover, this technique allows for analysis of the direct effects of specific molecules on the secretory pathway because the early signaling events elicited by receptor activation are effectively bypassed. As such, the use of cell permeabilization has become the gold-standard to study inhibitory peptides (4,20), toxins (14), pharmacological agents (11,12), dominant negative protein constructs (15,31,32), and to screen for novel cytosolic regulatory proteins (41) that modulate membrane fission and fusion. In addition to secretory studies, permeabilized cells have been used to investigate a variety of cellular functions including Ca2+-signaling and G-protein coupled receptor regulation (3,16)
Cholesterol binding cytolytic agents such as a-toxin, SLO and PFO have been extensively utilized in isolated pancreatic acinar cells (5,10,12,13,17-29,35,39-41). These toxins provide a controlled pore size creating selectivity of what can enter and leak out of the cells following permeabilization (12,23,35).
Alpha-toxin is purified from the bacterium Staphylococcus aureus and forms heptameric units upon binding cholesterol in the cell membrane. The polymerized structure is a transmembrane beta-barrel with a pore diameter of 1-2 nm (2). These pores are relatively small creating an approximate molecular weight cut off of 2-4 kDa (11). Padfield and Panesar have extensively utilized α-toxin in isolated acinar cells demonstrating its advantages for studying the effects of low molecular weight molecules including Mg-ATP, GTP-γ-S and aluminum fluoride in the secretory pathway (23,26,27). Because acini lose only small amounts of cytosolic molecules, they remain more highly responsive to secretory stimulation for extended periods of time in comparison to SLO and PFO, which effectively deplete cytosolic proteins within 10-15 following permeabilization (12,23,35). Alpha-toxin is available through Sigma (H9395) and must be used immediately after reconstituting the lyophilized powder.
SLO is purified from Streptococcus A, C and G. Following cholesterol binding, SLO polymerizes to form a transmembrane beta barrel with a pore size approximately 30 nm in diameter. Due to the larger pore size than that of a-toxin, SLO can be used for introduction of soluble polypeptides and proteins as large as immunoglobulins into cells. SLO has been used successfully by many labs studying secretion in isolated pancreatic acini (5,6,10,12,13,17-29,35) as well as Ca2+-uptake into intracellular stores (3) and regulator of G-protein signaling (RGS) function (16). Likely reflecting its instability following purification, it is difficult to obtain uniform activity across different batches of SLO, making reproducibility between experiments challenging. Moreover, once the lyophilized powder is reconstituted, it must be used immediately and cannot be aliquoted and/or frozen for later use. Conversely, recombinant SLO is highly pure and more stable, providing consistent results between experiments. However, recombinant SLO is much more expensive then the purified form. Recombinant SLO is available from Abcam (ab63978, $16/µg) and Aalto Bioreagents Ltd. (AW 6093-b, pricing unavailable online). Purified SLO can be purchased from Sigma (S5265, $5.50/KU).
PFO is produced in Clostridium perfringens and, like SLO, forms a transmembrane beta barrel pore of approximately 25 nm diameter (33). We have used recombinant PFO successfully in pancreatic acinar cells to study the role of cysteine string protein (40), various SNARE molecules (39) and complexin 2 (7) on acinar secretion. A His-tagged-PFO fusion protein expression plasmid was generously provided to our laboratory from AP Hueck and AE Johnson (8,9). This construct is a cysteine-less derivative of PFO in which cysteine 459 is replaced by alanine and as such does not require treatment with a thiol reducing agent for activation. The PFOcys459/ala mutation does not significantly affect hemolytic activity (33). Recombinant PFO is easily purified from bacteria by Co2+-affinity chromatography and can be aliquoted and stored at -80 oC for prolonged periods.
1. Materials and buffer solutions
1.1 Equipment
- Tabletop or other refrigerated preparative centrifuge.
- Water-bath style incubator capable of holding temperature at 37 oC.
- pH meter Note: The amount of free Ca2+ in these assay buffers is dependent on pH so it is imperative to have a well functioning, properly calibrated pH meter.
- A Chelator Software program to calculate the amount of free Ca2+ in the assay: refer to this web address for a free program http://www.stanford.edu/~cpatton/downloads.htm. Select the CaMgATPEGTA program. Be sure to include the Mg2+ concentrations from MgCl2 and Mg-ATP (you also need to include ATP alone) and base your calculations on the final concentration of each buffer component in the assay, NOT the stock solutions. A detailed description of “N = ionic strength” is provided by the author. For our assays we typically use N = 0.165
1.2 Stock Solutions
- Typically, the agents to be tested in permeabilized acini including recombinant proteins, peptides, toxins, immunoglobulins etc. are obtained in a minimal stock concentration of 1.5 mg/ml (see Note 1) in either 1X PBS (pH 7.4) or ideally Permeabilization buffer.
- Recombinant PFO is purified and stored in 25 μl aliquots at approximately 17.5 μM in 1X PBS. The molecular mass of recombinant PFO = 57,000 g/mol (1 mg/ml = 17.5 µM). If using purified SLO see point 3 in section in section 1.3 below.
- Stock solutions to prepare Permeabilization buffer:
- CaCl2 - 380 mM
- Na-EGTA (pH 8.0) - 200 mM
- Mg-ATP - 400 mM
- MgCl2 - 400 mM
(store all at 4 oC)
1.3 Buffers
- Acinar buffer: 139 mM Potassium Glutamate, 20 mM Pipes, 0.1 mg/ml soybean trypsin inhibitor (SBTI), 1.0 mg/ml BSA, pH 6.6. We typically prepare 200 ml of this buffer per experiment.
- Permeabilization buffer (Perm buffer): To approximately 95 ml of the above Acinar buffer add aliquots of the stock solutions listed above in section 1.2 point 3 to yield 4 mM EGTA, 1.78 mM MgCl2 and 2 mM Mg-ATP. Bring up to 100 ml final volume using Acinar buffer and re-pH to 6.6.
- Perm buffer containing SLO/PFO: (MADE IMMEDIATELY PRIOR TO USE) To 10 ml of the above Perm buffer add recombinant PFO to a final concentration of 35 nM. If using lyophilized SLO purified from Streptococci, dissolve the powder in Perm buffer immediately before use. We previously used SLO at a final concentration of 0.5 U/ml (33). Once dissolved, the SLO loses activity quickly. It cannot be aliquoted for storage. It is not necessary to readjust the pH of the buffer once the PFO or SLO have been added.
- Perm buffer with CaCl2: Using a free software program (see point 4, section 1.1 above) calculate the amount of CaCl2 to be added to the Perm buffer to give the desired final concentration of free Ca2+, and then add double that amount from the CaCl2 stock solution. Re-pH to 6.6. You must add double the calculated amount of Ca2+ at this stage because the concentration will be diluted by one half when added to the acinar cells suspended in Perm buffer alone (see step 5 of section 2.2 below).
1.4 Amylase Assay
- Phadebas may be ordered online at: http://www.phadebas.com/
- Phadebas assay buffer: 0.05M NaCl, 0.02 M NaH2PO4 and 0.02% Sodium Azide, pH 7.4.
2. Methods
2.1 Isolation of acinar cells
- It is necessary to finesse your organ digestion so the acinar cells are in small clumps (only 1-2 layers of cells in depth and groups of around 4-10 cells). This allows for “even binding” of the cytolytic agents to acini. Please refer to the entry by John Williams for purification of acini.
- In a typical permeabilization assay only small aliquots of cells are needed. After isolation, suspend 0.5 ml of packed acini in 35 ml of a physiological buffer such as DMEM or a HEPES buffered salt solution containing glucose and amino acids. The buffer should be supplemented with 1.0 mg/ml BSA and 0.1 mg/ml SBTI. Place acini in a 250 ml polycarbonate Erlenmeyer flask and fill the head space with O2. Allow acini to “rest” at 37oC for 1 hour, gently swirling the flask every 5 minutes to keep the cells suspended. Resting the cells is essential. If the assay is started immediately after isolation the cells will give a blunted response. The density of cells in the buffer while resting is also important; if too dense, acini will not respond as robustly when stimulated.
2.2 Permeabilization assay
- Remove 4 ml of cells, gently pellet, and resuspend in 10 ml of ice cold Perm buffer containing freshly prepared PFO or SLO in a small Erlenmeyer flask. KEEP THE FLASK OF CELLS ON ICE FOR 10 min, gently swirling the flask every 1-2 min to keep the cells suspended in solution. This step in the experiment must be done at 4oC as it is the “binding” step in which the PFO/SLO binds to the plasma membrane of the cell. Pore formation occurs upon warming cells to 37 oC (5,6).
- Transfer the cells to a prechilled 15 ml polycarbonate tube and gently pellet the cells (4 oC, </= 250 x g, 10-20 sec). Remove the supernatant and immediately return the pellet to the ice bath. Resuspend the cells (which now have PFO/SLO bound to them) in ice cold Perm buffer that DOES NOT contain Ca2+ or PFO and store them in a polycarbonate Erlenmeyer flask. This step of washing out unbound SLO/PFO at 4 oC from the assay is also important; if not removed, unbound SLO/PFO will enter the cells and permeabilize intracellular organelles.
- Prepare 1.5 ml microcentrifuge tubes for each of the treatment groups in the experiment with the necessary amount of protein/peptide (or chemical agent of interest) and arrange on racks that are submerged in the ice bath. Here the test agent is added anticipating a final assay volume of 400 µl (see Note 2). Also include 2 – 4 tubes that will be used to measure total cellular amylase.
- Using a 200 µl pipet with the tip trimmed (to prevent cell-shearing during aliquoting), aliquot 200 µl of the acinar suspension to each microfuge tube in a random manner (including the tubes that will be used to measure total cellular amylase). It is imperative to continually and gently swirl the flask containing the cells during aliquoting to ensure an equal distribution of cells in each tube. It is also imperative to maintain cells at 4 oC during aliquoting because raising the temperature will cause the SLO/PFO to activate.
- Before continuing on with the assay, using a tabletop centrifuge, pellet the 2-4 aliquots of cells to be used for measuring total cellular amylase. Draw off the supernatant without disrupting the pellet. The cell pellets can be kept on ice until the amylase assay is performed, at which time, they are briefly (5 sec) sonicated in 200 µL of water. Cut the samples 1:10 with water before amylase analysis. We typically sonicate the pellets immediately after aliquoting and store them on ice until analysis.
- 2.2A Preincubation with test agents
- At this point you can either continue on to step 7 below or incubate the cells in a 37 oC waterbath for 15 min to allow the permeabilization process to begin in the presence of the added test agent. If this preincubation step is necessary, the reagent should be added at the proper final concentration initially.
- Remove the tubes from the 37 oC water bath directly to the ice bath and then add the 200 µL of Perm buffer +/- Ca2+. Also include enough of the test agent to maintain its final concentration in the total assay volume of 400 µl. Then transfer all tubes at once back to the 37oC waterbath for 30 min. Continue on to step 8.
- Add 200 ml of ice cold Perm buffer +/- Ca2+ as indicated to each microfuge tube (still on ice); gently invert each to mix and transfer all tubes at once into 37 oC water-bath for 30 min. During the 30 min incubation it is necessary every 1-2 minutes to gently invert each microcentrifuge tube to resuspend the cells in solution.
- At the end of the 30 min incubation, remove all microcentrifuge tubes at once to an ice bath. Centrifuge samples at 14,000 rpm for 30 sec at 4 oC and collect the supernatant to be used in the amylase assay.
2.3 Amylase Assay
- Follow entry by Williams for use of Phadebas to measure amylase activity and calculate the cellular secretion.
3. Notes
- Because the agents to be tested will contribute to the total final volume of the assay, it is important to use a minimum of 1.5 mg/ml stock solution to avoid diluting the buffer components. There can sometimes be complications using a stock solution with a concentration above 3.5 mg/ml (ie aggregation or precipitation) but this is dependent on the physical properties of the peptide or protein and should be determined on an individual basis. Likewise, control samples not receiving the test-agents should receive equal amounts of vehicle.
- Proteins and small peptides are typically added at a range of 10-300 µg/ml yielding final concentrations in the µM range. Reagents (Ca2+, GTPγs, phorbol esters, cAMP, etc.) should be added paying attention to the desired final concentration in a final assay volume of 400 µL.
4. Anticipated Results
The rate and extent of permeabilization can be followed by analyzing the accumulation of soluble proteins in the extracellular space. This is typically achieved by measuring activity of a cytosolic enzyme such as lactate dehydrogenase (12) or by immunoblotting specific proteins of interest (35), although this later analysis usually requires trichloroacetic acid precipitation of the media to concentrate the protein. Additionally, the introduction of proteins into the cytoplasm may also be analyzed by immunofluorescence microscopy. Figure 1 shows the accumulation of recombinant green fluorescent protein (GFP) into acini following 30 min incubation with permeabilized acini. The apparent accumulation of GFP within the apical aspects of the acini is due in part to our demonstration of a single z-plane through the acini together with the rapid loss of GFP from the periphery of the cells following removal of the incubation medium and washing in GFP-free buffer immediately prior to analysis. This same approach can be used with antibodies or fluorescently-labeled binding proteins that recognize organelle-specific proteins or phospholipids. Such studies may be conducted with or without fixation prior to fluorescence analysis and may help in building a case for the specificity of a given reagent in regulating a distinct cellular activity. For example, an agent that modulates secretion may be expected to localize to secretory granules or sites of exocytosis along the plasma membrane.
Permeabilized acini remain highly sensitive to changes in the free Ca2+ concentration, although there is some variability reported between different laboratories. Most investigators report a concentration-dependent increase in amylase release in a range of 0.1 to 30 µM free Ca2+. Kitagawa et al. (12) and Edwardson et al. (6) reported maximal secretion occurred at 3 µM free Ca2+ and Thomas et al. (35) similarly showed 10 µM free Ca2+; however, Stecher et al. (34) reported 30 µM free Ca2+ elicited the maximal secretory response. Because of these variations it is important that once established, the Ca2+ sensitivity of your assay should be evaluated. The use of 3-10 μM free Ca2+ is sufficient for initial experiments. Of note, basal Ca2+ is achieved by omitting CaCl2 in the assay system and is typically reported as [Ca2+] 1 nM.
In addition to evaluating the Ca2+-sensitivity of your assay system, identifying the concentration-dependent effect of Ca2+ on the ability of a given test-agent to modulate secretion can also be quite useful. Shown in Fig 2A, is the effect of the protein CRHSP-28 (aka TPD52) to augment secretion over a range of Ca2+ concentrations in permeabilized acini. Note that the ability of CRHSP-28 to enhance secretion is most pronounced at sub-maximal levels of Ca2+. Once the optimal Ca2+ level has been established, a concentration-response and time course may be determined for the test agent (Fig 2B and 2C). We have found that recombinant proteins and peptides often function in a range within 10 – 400 µg/ml which depending on the molecular size, is roughly µM levels.
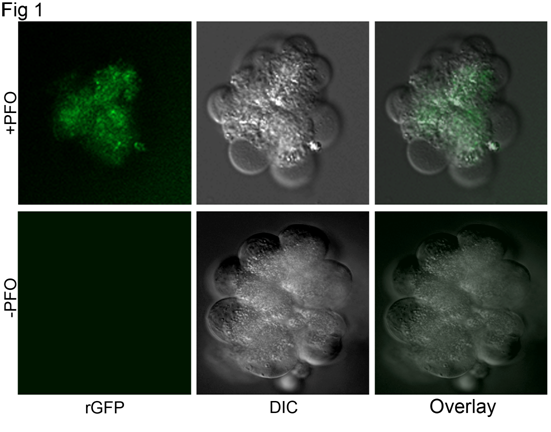
Figure 1. Purified acini were suspended in a permeabilization buffer containing (in mM) 20 PIPES (pH 6.6), 139 K+-glutamate, 4 EGTA, 1.78 MgCl2, 2 Mg-ATP, 0.1 mg/ml soybean trypsin inhibitor, 1 mg/ml bovine serum albumin, and 36 nM PFO. The PFO was allowed to bind to the cells on ice for 10 min and was then removed by washing at 4 °C in the same buffer without PFO. Acini were aliquoted into microcentrifuge tubes (80 µL/tube) containing 25 μg of rGFP and brought to a final volume of 400 μL with permeabilization buffer without PFO. Cell suspensions were immersed for 30 min in a 37 °C water bath and incubated with gentle mixing every 2 min. At the end of the incubation period, cells were immediately placed in an ice bath and then briefly centrifuged at 12,000 × g to pellet. The excess media was drawn off, cells were resuspended in 50 μL of 1X PBS and then aliquoted onto a coverslip. Brightfield images were captured with excitation and emission filters appropriate for GFP using a Nikon Eclipse TE2000 microscope, a PlanApo 20x objective with a numerical aperture of 0.75, and a Hamamatsu Orca camera. Images were captured using Volocity software and were processed for publication with Photoshop software.
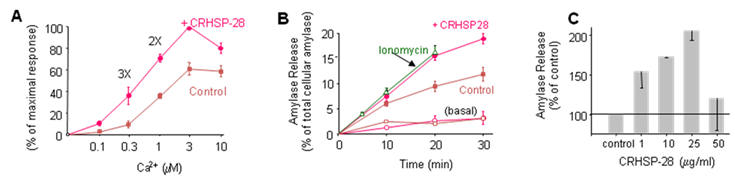
Fig 2. CRHSP-28 reconstitutes Ca2+-stimulated secretion A) Streptolysin-O permeabilized acini were incubated as control or with recombinant CRHSP-28 (25μg/ml) and amylase secretion in response to various Ca2+ concentrations were determined. Note CRHSP-28 increased secretion by 2 and 3 fold at sub-maximal Ca2+ levels B) Streptolysin-O permeabilized acini were incubated as control or with recombinant CRHSP-28 (25μg/ml). Amylase secretion was measured over time in response to basal (<10 nM) or stimulatory (10 mM) concentrations of free Ca2+. Amylase secretion from intact cells stimulated with ionomycin (2μM) is included for comparison. C) Concentration dependent effects of CRHSP-28 measured while stimulating cells with 1 μM free Ca2+. This data was reproduced from ref 35.
In addition to Ca2+, secretion from permeabilized acini is also sensitive to GTPγS, phorbol esters and cAMP (12,13). Because GTPγS activates both small and large G-protein signaling pathways, AlF3, which inhibits heterotrimeric G-protein activation, has been used in a-toxin permeabilized acini to distinguish between these classes of molecules (27,28). Additionally, the ATP-dependence of an agent to modulate secretion is difficult to demonstrate because the energy consumed during exocytosis and membrane fusion is derived from conformational changes in SNARE proteins on secretory granules and plasma membrane when they are in brought in close contact. ATP is subsequently consumed by a chaperone complex composed of NSF and SNAP which “unzips” the SNARE complex post-fusion.
Protein Purification
Production of recombinant proteins for use in permeabilized cells can pose some difficulties depending on the physical properties of the molecule. If purified from a GST-fusion construct and cleaved with thrombin, the thombin may be inhibited by addition of benzamidine. The benzamidine may be removed by dialysis or buffer exchange. Alternatively, immobilized benzamidine (available through GE Heathcare) can be used for thrombin removal. We have found that thrombin activity is lost during incubation for GST-protein cleavage and in control experiments has no effect on secretion even without removal. If imidizole is used to elute His-tagged proteins from Ni- or Co2+-affinity columns it is imperative that it be completely removed by dialysis or buffer exchange. We have found that imidizole greatly alters secretory activity.
Correct folding of recombinant proteins may also pose some problems. We found in one case that biological activity was best achieved by thawing a particular protein and keeping it in the refrigerator for at least a day provided the best results. Others have used baculovirus expression of proteins in SF9 cells which retain a high translational capacity. Under these conditions cytosolic extracts can be analyzed directly without purification and the results compared to extracts from cells infected with a control baculovirus.
In selecting a suitable control for a protein of interest many labs rely on the fact that the assay contains ample amounts of BSA and SBTI (1 mg/ml and 0.1 mg/ml respectively). The use of protein mutation, either in the form of truncation or site specific alterations, is ideal not only for investigating the molecular determinants of a particular effect but also providing an internal control to account for any off target effects of the assay. This is especially true for loss of function mutations.
Protocol Variations
The process of warming the cells in the presence of elevated Ca2+ to initiate secretion is very convenient; however, it potentially has some foreseen and unforeseen drawbacks. Two examples come to mind: The concentration of free Ca2+ in the assay is mildly dependent on temperature suggesting that free ion concentrations are not stable during the warming period. Additionally, chilling cells to 4 oC is known to rapidly depolymerize microtubules, which then quickly repolymerize with cell warming. It is conceivable that if the analysis performed is impacted by microtubule dynamics significant fluctuations in activity may occur. As an alternative it is possible to chill the cells for PFO/SLO binding. Cells may then be added sequentially to a 37 oC water bath for preincubation in basal Ca2+ and then sequentially stimulated by addition Ca2+ containing buffer.
References
- Aikawa Y, and Martin TFJ. ARF6 regulates a plasma membrane pool of phosphatidylinositol (4,5) bisphosphate required for regulated exocytosis. J Cell Biol 162(4): 647-659, 2003. PMID: 12925709
- Bhakdi S, Bayley H, Valeva A, Walev I, Walker B, Kehoe M and Palmer M. Staphylococcal alpha-toxin, streptolysin-O, and Escherichia coli hemolysin: prototypes of pore-forming bacterial cytolysins. Arch Microbiol. 165(2):73-9, 1996. PMID: 8593102
- Bruce JI, Yule DI and Shuttleworth TJ. Ca2+-dependent protein kinase--a modulation of the plasma membrane Ca2+-ATPase in parotid acinar cells. J Biol Chem. 277(50):48172-81, 2002. PMID: 12368283
- Chen YA, Scales SJ, Jagath JR, and Scheller RH. A discontinuous SNAP-25 C-terminal coil supports exocytosis. J Biol Chem 276: 28503-28508, 2001. PMID: 11373287
- Cher DJ, Padfield PJ and Jamieson JD. Amylase release from streptolysin O permeabilized fetal pancreatic acini. Am J Physiol. 262(4 Pt 1):G719-26, 1992. PMID: 1373567
- Edwardson JM, Vickery C, Christy LJ. Rat pancreatic acini permeabilised with streptolysin O secrete amylase at Ca2+ concentrations in the micromolar range, when provided with ATP and GTP gamma S. Biochim Biophys Acta. 1053(1):32-6. 1990 PMID: 1694696
- Falkowski MA, Thomas DD and Groblewski GE. Complexin 2 modulates vesicle-associated membrane protein (VAMP) 2-regulated zymogen granule exocytosis in pancreatic acini. J Biol Chem. 285(46):35558-66, 2010. PMID: 20829354
- Heuck AP, Tweten RK and Johnson AE. ß-Barrel Pore-Forming Toxins: Intriguing Dimorphic Proteins. Biochemistry, 40 (31) 9065–9073, 2001. PMID: 11478872
- Heuck AP, Tweten RK and Johnson AE. Assembly and topography of the prepore complex in cholesterol-dependent cytolysins. J Biol Chem. 278(33):31218-25, 2003. PMID: 12777381
- Jena BP, Padfield PJ, Ingebritsen TS and Jamieson JD. Protein tyrosine phosphatase stimulates Ca(2+)-dependent amylase secretion from pancreatic acini. J Biol Chem. 266(27):17744-6, 1991. PMID: 1717440
- Kaspar KM, Thomas DD, Taft WB, Takeshita E, Weng N and Groblewski GE. CaM kinase II regulation of CRHSP-28 phosphorylation in cultured mucosal T84 cells. Am J Physiol Gastrointest Liver Physiol. 285(6):G1300-9, 2003. PMID: 12893633
- Kitagawa M, Williams JA, De Lisle RC. Amylase release from streptolysin O-permeabilized pancreatic acini. Am J Physiol. 259(2 Pt 1):G157-64, 1990. PMID: 1696432
- Kitagawa M, Williams JA, De Lisle RC. Interactions of intracellular mediators of amylase secretion in permeabilized pancreatic acini. Biochim Biophys Acta. 1073(1):129-35, 1991. PMID: 1703790
- Li Q, Ho CS, Marinescu V, Bhatti H, Bokoch GM, Ernst SA, Holz RW, and Stuenkel EL. Facilitation of Ca2+-dependent exocytosis by Rac1-GTPase in bovine chromaffin cells. J Physiol 550: 431-445, 2003. PMID: 12754309
- Lmai A, Yoshie S, Nashida T, Shimomura H, and Fukuda M. The small GTPase Rab27B regulates amylase release from rat parotid acinar cells. J Cell Sci 117: 1945-1953, 2004. PMID: 15039459
- Luo X, Ahn W, Muallem S and Zeng W. Analyses of RGS protein control of agonist-evoked Ca2+ signaling. Methods Enzymol. 389:119-30, 2004. PMID: 15313563
- Muallem S, Kwiatkowska K, Xu X, and Yin HL. Actin filament disassembly is a sufficient final trigger for exocytosis in nonexcitable cells. J Cell Biol 128; 589-598, 1995. PMID: 7860632
- Ohnishi H, Ernst SA, Wys N, McNiven M, and Williams JA. Rab3D localizes to zymogen granules in rat pancreatic acini and other exocrine glands. Am J Physiol, 271; G531-G538, 1996. PMID: 8843780
- Ohnishi H, Mine T, Shibata H, Ueda N, Tsuchida and T, Fujita T. Involvement of Rab4 in regulated exocytosis of rat pancreatic acini. Gastro. 116; 943-952, 1999. PMID: 10092317
- Ohnishi H, Ernst S, Yule DI, Baker CW, and Williams JA. Heteromeric G-protein Gq/11 localized on pancreatic zymogen granules is involved in calcium-regulated amylase secretion. J Biol Chem. 272; 16056-16061, 1997. PMID: 9188511
- O'Sullivan AJ and Jamieson JD. Activation of protein kinase C is not an absolute requirement for amylase release from permeabilized rat pancreatic acini. Biochem J. 285 ( Pt 2):597-601,1992. PMID: 1379047
- O'Sullivan AJ and Jamieson JD. Protein kinase A modulates Ca(2+)- and protein kinase C-dependent amylase release in permeabilized rat pancreatic acini. Biochem J. 287 ( Pt 2):403-6, 1992. PMID: 1280101
- Padfield PJ and Panesar N. Ca(2+)-dependent amylase secretion from SLO-permeabilized rat pancreatic acini requires diffusible cytosolic proteins. Am J Physiol. 269(5 Pt 1):G647-52, 1995. PMID: 7491954
- Padfield PJ, Panesar N, Henderson P and Baldassare JJ. Differential effects of G-protein activators on 5-hydroxytryptamine and platelet-derived growth factor release from streptolysin-O-permeabilized human platelets. Biochem J. 314 ( Pt 1):123-8, 1996. PMID: 8660272
- Padfield PJ and Panesar N. MgATP acts before Ca(2+) to prime amylase secretion from permeabilized rat pancreatic acini. Am J Physiol. 273(3 Pt 1):G655-60, 1997. PMID: 9316469
- Padfield PJ and Panesar N. Cholecystokinin octapeptide inhibits Ca(2+)-dependent amylase secretion from permeabilized pancreatic acini by blocking the MgATP-dependent priming of exocytosis. Biochem J. 330 (Pt 1):329-34, 1998. PMID: 9461527
- Padfield PJ and Panesar N. The two phases of regulated exocytosis in permeabilized pancreatic acini are modulated differently by heterotrimeric G-proteins. Biochem Biophys Res Commun. 245(2):332-6, 1998. PMID: 9571150
- Padfield PJ, Ding TG and Jamieson JD. Ca(2+)-dependent amylase secretion from pancreatic acinar cells occurs without activation of phospholipase C linked G-proteins. Biochem Biophys Res Commun. 174(2):536-41, 1991. PMID: 1899567
- Padfield PJ, Balch WE and Jamieson JD. A synthetic peptide of the rab3a effector domain stimulates amylase release from permeabilized pancreatic acini. Proc Natl Acad Sci U S A. 89(5):1656-60, 1992. PMID: 1371881
- Palmer M, Harris R, Freytag C, Kehoe M, Tranum-Jensen J, and Bhakdi S. Assembly mechanism of the oligomeric streptolysin O pore: the early membrane lesion is lined by a free edge of the lipid membrane and is extended gradually during oligomerization. EMBO J. 17(6): 1598–1605, 1998. PMID: 9501081
- Polgar J, Chung SH, and Reed GL. Vesicle associated membrane protein 3 (VAMP-3) and VAMP-8 are present in human platelets and are required for granule secretion. Blood 100: 1081-1083, 2002. PMID: 12130530
- Quetglas S, Iborra C, Sasakawa N, De Haro L, Kumakura K, Sato K Le C, and Seagar. Calmodulin and lipid binding to synaptobrevin regulates calcium dependent exocytosis. EMBO J 21: 3970-3979, 2002. PMID: 12145198
- Shepard LA, Heuck AP, Hamman BD, Rossjohn J, Parker MW, Ryan KR, Johnson AE and Tweten RK. Identification of a membrane-spanning domain of the thiol-activated pore-forming toxin Clostridium perfringens perfringolysin O: an alpha-helical to beta-sheet transition identified by fluorescence spectroscopy. Biochemistry 37(41):14563-74, 1998. PMID: 9772185
- Stecher B, Ahnert-Hilger G, Weller U, Kemmer TP, Gratzl M. Amylase release from streptolysin O-permeabilized pancreatic acinar cells. Effects of Ca2+, guanosine 5'-[gamma-thio]triphosphate, cyclic AMP, tetanus toxin and botulinum A toxin. Biochem J. 283 (3):899-904. 1992. PMID: 1375453
- Thomas DDH, Taft WB, Kaspar KM and Groblewski GE. CRHSP-28 regulates Ca(2+)-stimulated secretion in permeabilized acinar cells. J Biol Chem. 276(31):28866-72, 2001. PMID: 11384973
- Tucker WC, Edwardson JM, Bai HK, Martin TF, and Chapman ER. Identification of synaptotagmin effectors via acute inhibition of secretion from cracked PC12 cells. J Cell Biol.162, 199-209, 2003. PMID: 12860971
- Tweten RK. Cholesterol-Dependent Cytolysins, a family of versatile pore-forming toxins. Infect Immun. 73(10): 6199–6209, 2005. PMCID: PMC1230961
- Wang L, Ungermann C, and Wickner W. The docking of primed vacuoles can be reversibly arrested by excess Sec17p (alpha-SNAP). J. Biol. Chem. 275, 22862-22867, 2000. PMID: 10816559
- Weng N, Thomas DDH and Groblewski GE. Pancreatic acinar cells express vesicle-associated membrane protein 2- and 8-specific populations of zymogen granules with distinct and overlapping roles in secretion. J Biol Chem. 282(13):9635-45, 2007. PMID: 17272274
- Weng N, Baumler MD, Thomas DDH, Falkowski MA, Swayne LA, Braun JE and Groblewski GE. Functional role of J domain of cysteine string protein in Ca2+-dependent secretion from acinar cells. Am J Physiol Gastrointest Liver Physiol. 296(5):G1030-9, 2009. PMID: 19282376
- Walent JH, Porter BW and Martin TF. A novel 145 kd brain cytosolic protein reconstitutes Ca(2+)-regulated secretion in permeable neuroendocrine cells. Cell. 70(5):765-75, 1992. PMID: 1516133