Entry Version:
Citation:
Pancreapedia: Exocrine Pancreas Knowledge Base, DOI: 10.3998/panc.2015.17
Attachment | Size |
---|---|
![]() | 913.33 KB |
1. What are the functions of the pancreas?
The pancreas has both exocrine and endocrine function. This chapter is devoted to the exocrine functions of the pancreas. The exocrine function is devoted to secretion of digestive enzymes, ions and water into the intestine of the gastrointestinal (GI) tract. The digestive enzymes are necessary for converting a meal into molecules that can be absorbed across the surface lining of the GI tract into the body. Of note, there are digestive enzymes secreted by our salivary glands, stomach and surface epithelium of the GI tract that also contribute to digestion of a meal. However, the exocrine pancreas is necessary for most of the digestion of a meal and without it there is a substantial loss of digestion that results in malnutrition.
The ions and water secreted are also critical for pancreas function as the resultant fluid is necessary to carry the digestive enzymes through the pancreatic ductal system into the intestine. In addition, the pH of the pancreatic secretions is alkaline due to a very high concentration of NaHCO3 in the fluid. A major function of the NaHCO3 is to neutralize the acidic pH of the gastric contents delivered to the intestine from the stomach. A neutral pH in the intestinal lumen is necessary for normal digestion and absorption.
This chapter presents processes underlying the functions of the exocrine pancreas with references to how specific abnormalities of the pancreas can lead to disease states.
2. Where is the pancreas located?
The illustration in Figure 1 demonstrates the anatomical relationships between the pancreas and organs surrounding it in the abdomen. The regions of the pancreas are the head, body, tail and uncinate process (Figure 2). The distal end of the common bile duct passes through the head of the pancreas and joins the pancreatic duct as it enters the intestine (Figure 2). Because the bile duct passes through the pancreas before entering the intestine, diseases of the pancreas such as a cancer at the head of the pancreas or swelling and/or scarring of the head of the pancreas from pancreatitis can block the bile duct system resulting in jaundice in a patient. As seen in Figure 1, the pancreas is situated deep in the abdomen and thus is usually protected from trauma. However, occasionally severe trauma as might occur from a steering wheel in an auto accident can “crush” the pancreas against the vertebral column that sites just behind the pancreas. Such an injury can result in pancreatitis.
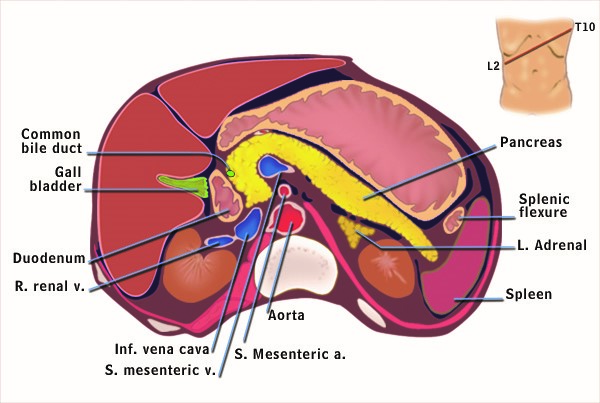
Figure 1. Cross sectional anatomy of the abdomen.This cartoon represents the anatomical features of a “slice” of the abdomen at the level depicted in the upper right hand corner of the figure. Anterior to the pancreas are the stomach, colon, omentum and loops of small intestine. Posterior to the pancreas are the portal vein, inferior vena cava, aorta, superior mesenteric artery and vein, kidneys and vertebrae. The distal common bile duct passes through the head of the pancreas. Adapted from Gorelick F, Pandol, SJ, Topazian M. Pancreatic physiology, pathophysiology, acute and chronic pancreatitis. Gastrointestinal Teaching Project, American Gastroenterological Association. 2003.

Figure 2. Anatomic regions and blood vessels of the pancreas.The anatomic regions are the head, neck, body and tail. Behind the pancreas lie the portal vein, inferior vena cava, aorta and the superior mesenteric artery and vein. The distal common bile duct passes through the head of the pancreas. Adapted from Gorelick F, Pandol, SJ, Topazian M. Pancreatic physiology, pathophysiology, acute and chronic pancreatitis. Gastrointestinal Teaching Project, American Gastroenterological Association. 2003.

Figure 3. The exocrine and endocrine pancreas.The pancreas is divided into an exocrine portion (acinar and duct tissue) and an endocrine portion (islets of Langerhans). The exocrine portion, comprising 85% of the mass of the pancreas is composed of acinar tissue which synthesizes, stores and secretes digestive enzymes; and ductal tissue which secretes water and NaHCO3, The endocrine portion secretes its hormones into the blood stream. The blood flow from the endocrine pancreas passes to the exocrine pancreas before entering the general circulation. Adapted from Gorelick F, Pandol, SJ, Topazian M. Pancreatic physiology, pathophysiology, acute and chronic pancreatitis. Gastrointestinal Teaching Project, American Gastroenterological Association. 2003.
The pancreas receives blood from two major arterial supplies (Figure 2). Because of the dual blood supply, ischemia to the pancreas from vascular obstruction is uncommon. Venous blood leaving the pancreas is via the splenic vein as the spleen is contiguous to the tail of the pancreas; this vein empties into the portal vein which carries blood from the GI tract to the liver. Diseases such as pancreatitis and pancreatic cancer can cause thrombosis and/or blockage of the draining of the spleen and pancreas. In this case the spleen becomes engorged with blood causing its enlargement which results in several undesirable consequences.
The pancreas is connected by the nervous system containing both sensory neurons and effector neurons. The sensory neurons function for pain perception so that diseases of the pancreas cause significant pain while the effector neurons are involved in regulating the secretions of both the exocrine and endocrine pancreas.
Although this chapter is devoted to the exocrine pancreas, it is important to point out that there are important inter-relationships between the endocrine (Islets of Langerhans) and exocrine pancreas. As shown in Figure 3 the blood flow from the endocrine pancreas enters the capillaries of the exocrine tissue surrounding each of the islets before entering the general circulation (4). Thus, the exocrine pancreatic tissue surrounding the islets is exposed to very high concentrations of hormones such as insulin coming from the islets. Normally, the islet hormones promote the function of the exocrine gland including the regulation of digestive enzyme synthesis (13, 14, 32). However, this arrangement may also be detrimental in patients with diabetes accounting for a greater risk of pancreatitis and pancreatic cancer.
3. What are the cells of the exocrine pancreas?
The two major cell types of the exocrine pancreas are the acinar cell and the ductal cell. The acinar cells are formed into a unit called the acinus which is connected to the ductal system composed of ductal cells (Figure 3). The two types of cellular units are combined to direct digestive enzymes into the duodenum where digestion is initiated.
The acinar cells are specialized to synthesize, store, and secrete digestive enzymes into a lumen space that is connected to the ductal system (Figure 4). The acinar cell has receptors for hormones and neurotransmitters that regulate the secretion of digestive enzymes into the lumen space (40). The acinar cell contains abundant rough endoplasmic reticulum for digestive enzyme synthesis (Figure 4). Beneath the lumen are zymogen granules, the storage depot of digestive enzymes.
The duct cells form the ductal system and contain abundant mitochondria necessary for energy products (ATP) needed for ion transport (see Figure 4). Two other types of cells of importance for the exocrine pancreas are the centroacinar cell and the stellate cell. Centroacinar cells have ductal cell characteristics and are also likely stem cells for populating the different cell types for the pancreas.
The pancreatic stellate cell (PaSC) is important because of its role in pathologic states (1-3, 23, 26, 36). The stellate cell is a very slender star-shaped (hence the name stellate) cell that drapes itself around the acini, ducts and the Islets of Langerhans. In normal function PaSCs are involved in directing proper formation of the epithelial structures. In pathologic states such as chronic pancreatitis and pancreatic cancer the PaSC is transformed into a proliferating myofibroblastic cell type that synthesizes and secretes extracellular matrix proteins, pro-inflammatory cytokines and growth factors. In this transformed state PaSCs promote the inflammation and fibrosis of both chronic pancreatitis and pancreatic cancer that are key characteristics of these diseases.
4. How does the acinar cell operate?
The acinar cells of the pancreas produce more protein than any of our organs. As such, the cells have a highly developed and large endoplasmic reticulum (ER) system where the digestive enzymes and other proteins are synthesized (Figure 5). The ER also is a store for calcium which when released into the cytoplasm is the mediator of regulated secretion of stored digestive enzymes into the pancreatic ductal system (30).
Each protein molecule synthesized in the ER must undergo specific secondary modifications as well as folding in order for it to be properly transported to destination organelles such as Golgi where further processing takes place and zymogen granule for storage of digestive enzymes. The zymogen granule stores digestive enzymes that are released by exocytosis with stimulation by nerves and hormones activated during a meal. Also, the systems for both protein synthesis and processing must be able to adapt because of variation in the demand for protein synthesis as a function of diet; and because protein processing in the ER could be adversely affected by environmental factors such as alcohol, smoking, altered metabolism and even medications.
5. What digestive enzymes are synthesized by the acinar cell?
The human pancreas and its acinar cells have the largest capacity for protein synthesis of any organ in the human body. Much of the capacity is devoted to synthesis of the digestive enzymes that are secreted in the intestinal lumen where they are necessary for digesting and assimilating the nutrients in a meal. The enzymes fall into four classes- proteolytic, amylolytic, lipolytic, and nuclease digestive enzymes (5, 33, 39). Some of the enzymes are present in more than one form (e.g., cationic trypsinogen, anionic trypsinogen, and mesotrypsinogen). Many of the digestive enzymes are synthesized and stored in the acinar cells as pro-enzymes that have no enzymatic activity.

Figure 4. Ultrastructure of acinar and duct cells of the exocrine pancreas. The pancreatic acinar cell has prominent basally located rough endoplasmic reticulum for synthesis of digestive enzymes (and other proteins) and apically located zymogen granules for storage and secretion of digestive enzymes. The zymogen granules undergo exocytosis with stimulation of secretion. The secretion is into the lumen of the acinus formed by the apical surfaces of the acinar cells. Pancreatic duct cells contain abundant mitochondria for energy generation needed for its ion transport functions. The ductal cells also project microvilli into the luminal space. Adapted from Gorelick F, Pandol, SJ, Topazian M. Pancreatic physiology, pathophysiology, acute and chronic pancreatitis. Gastrointestinal Teaching Project, American Gastroenterological Association. 2003.

Figure 5. Electron micrograph of the pancreatic acinar cell.This electron micrograph shows the key cellular structures involved in synthesis, processing, and storage of digestive enzymes. On the left is the rough endoplasmic reticulum; in the middle is the Golgi complex; and on the right are zymogen granules. Adapted from Gorelick F, Pandol, SJ, Topazian M. Pancreatic physiology, pathophysiology, acute and chronic pancreatitis. Gastrointestinal Teaching Project, American Gastroenterological Association. 2003.
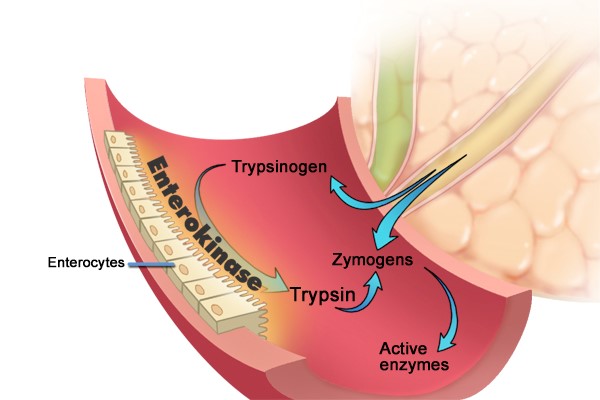
Figure 6. Intestinal digestive enzyme activation. Inactive proenzymes called zymogens enter the duodenum where enterokinase which is attached to the intestinal surface enzymatically cleaves trypsinogen activating it to trypsin. Trypsin, in turn, converts zymogens including trypsinogen itself to their activated enzyme forms through enzymatic cleavage. Adapted from Gorelick F, Pandol, SJ, Topazian M. Pancreatic physiology, pathophysiology, acute and chronic pancreatitis. Gastrointestinal Teaching Project, American Gastroenterological Association. 2003.
This is important as the active enzymes are capable of digesting the cell causing injury that can lead to pancreatitis. Other mechanisms to prevent these enzymes from potentially digesting the pancreas include the storage and packing the enzymes into acidic zymogen granules; and including trypsin activity inhibitors in the zymogen granules. Pro-enzymes are activated when they enter the duodenum.
As illustrated in Figure 6, activation of these enzymes takes place on the surface of the duodenal lumen, where a brush-border glycoprotein peptidase, enterokinase, activates trypsinogen by removing an N-terminal hexapeptide fragment of the molecule resulting in an enzymatically active form (5, 31, 39). The active form, trypsin, then catalyzes the activation of the other inactive proenzymes. Of note, many key digestive enzymes such α-amylase and lipase are present in the pancreas in their active forms. Presumably, these enzymes would not cause pancreatic cellular damage if released into the pancreatic cell/tissue because there is no starch or glycogen (substrates for α-amylase) or triglyceride (substrate for lipase) for these enzymes in pancreatic tissue. However, obesity may enhance pancreatitis as a result of increase triglyceride in and around the pancreas.
6. What do acinar cell digestive enzymes accomplish in the gastrointestinal tract?
Digestion of food occurs in the alimentary tract. Although the pancreatic acinar cell digestive enzymes are critical, they do not account for all of the processes needed from complete digestion and absorption of the nutrients in a meal.Examples of additional digestion include amylase secreted from the salivary glands that can accomplish up the 50% of the intraluminal digestion of starch and glycogen, especially in newborns. Further, the action of both salivary and pancreatic amylase is to hydrolyze every other junction between the glucose molecules of starch and glycogen. Thus, there remains products that need further digestion to single glucose molecules that can be absorbed.

Figure 7. Regulation of exocytosis. Digestive enzymes are stored in zymogen granules at the apical surface of the acinar cell. Regulated secretion occurs through exocytosis stimulated by neurohumoral agents. These agents include gastrin releasing peptide (GRP), cholecystokinin (CCK), acetylcholine (Ach), secretin and vasoactive intestinal polypeptide (VIP). Each acts to mediate secretion through interacting with its specific receptor. For example, specific interaction of CCK with its receptor (CCK1 receptor) leads to activation of intracellular signaling systems that mediate the exocytosis response. Interactions of GRP, CCK and Ach with their receptors lead to changes in intracellular calcium (Ca+2) which, in turn, mediates exocytosis. On the other hand, secretin and VIP increase intracellular cAMP which, in turn, mediates the exocytosis response. Of note, an increase in both calcium and cAMP simultaneously results in synergistic response. That is, the response with both is greater than the expected additive effect. Adapted from Gorelick F, Pandol, SJ, Topazian M. Pancreatic physiology, pathophysiology, acute and chronic pancreatitis. Gastrointestinal Teaching Project, American Gastroenterological Association. 2003.
The final processes of digestion of starch and glycogen involves enzymes attached to the surface of the small intestine which complete hydrolysis of the products of amylase digestion to glucose.
Then, glucose is transported across the intestinal absorptive epithelial cell by a Na+-coupled transport (11, 41). In the case of proteases, gastric secretion of pepsin accomplishes a small portion of protein digestion in the lumen of the alimentary tract and a complement of several pancreatic proteases (each catalyzing cleavage at specific sites in the meal protein) further act on protein to provide oligopeptides, di- and tri-peptides as well as amino acids. The oligopeptides, di- and tri-peptides are further digested by enzymes on the lumenal surface of the small intestine. Then free amino acids and some small peptides are transported across the intestinal mucosa by a group of Na+- and H+-coupled transporters (10).
Lipases are secreted mainly by the pancreas in contrast to amylase where there is a significant salivary contribution. There are lingual and gastric lipases but these contribute to fat digestion in only a minor fashion. Major lipases secreted by the pancreas are lipase (or triglyceride lipase) and prophospholipases. Pancreatic lipase hydrolyzes a triglyceride molecule to two fatty acid molecules released from carbons 1 and 3 and a monoglyceride with a fatty acid esterified to glycerol at carbon 2 (7). These components are then absorbed by specific transporters on the surface of the small intestinal mucosa.
7. How is secretion of digestive enzymes from the acinar cell regulated?
Digestive enzymes that are synthesized and stored in the zymogen granule are available for release into the lumen of the pancreatic acinus and passage through the pancreatic ductal system into the intestine. The process of transporting zymogen granule contents into the lumen of the acinus is called exocytosis (25). The regulation of release of the zymogen granule contents occurs through signals arriving at the acinar cell coming from both hormones and nerves that act through receptors on the cell resulting in an increase in intracellular messengers cyclic adenosine monophosphate (cyclic AMP) and ionic calcium (Ca+2) shown in Figure 7 (22).
8. What are the environmental and genetic stressors of the acinar cell that promote diseases of the pancreas?
The most common diseases of the exocrine pancreas are pancreatitis and pancreatic cancer. Gallstone disease uniquely underlies a large proportion of cases of pancreatitis. Alcohol abuse, smoking and diabetes are key risk factors in the epidemiology of both diseases (27, 42). How these environment factors mediate these disorders is incompletely understood. An important and unexplained observation is that only a small proportion of heavy drinkers, smokers and diabetics develop pancreatic diseases (29). Although the reason for the lack of development of pathology in the majority of those who have these disorders is unknown, it is likely that an adaptive and protective response is mounted to adjust the processes in the acinar cell to prevent failure of function and disease formation (18, 28).
Genetic causes of pancreatic diseases related to the acinar cell are due to mutations in the digestive enzymes. The best studied of these are mutations in cationic trypsinogen which result in inappropriate conversion of the pro-enzyme trypsinogen to active trypsin in the acinar cell (37, 38). As noted above, activation of digestive enzymes within the cell can lead to damage and disease. Mutations lead to both pancreatitis and, over time, to pancreatic cancer as a result of the chronic inflammatory process.
9. How does the pancreatic ductal system transport the digestive enzymes to the intestine?
The ductal system creates a large flow of fluid to carry to carry the acinar cell secretions to the intestine. The fluid is rich in bicarbonate (HCO3–) which is necessary to neutralize the gastric acid that enters into the intestine during a meal. The neutralization of gastric acid is necessary because the pancreatic enzymes have optimal activity at neutral pH. At the acid pH of gastric secretions pancreatic digestive enzymes are ineffective in digesting a meal. The water and bicarbonate secretion are created by a complex set of interacting ion transport pathways in the ductal cells as illustrated in Figure 8. As is discussed below, disorders of water and ion secretion lead to pancreatic tissue damage and exocrine failure as occurs in cystic fibrosis.
The regulation of water and ion secretions is largely mediated by the hormone secretin and the neurotransmitter acetylcholine stimulation of the pancreatic ductal cell. Binding of secretin to its receptor results in an increase in cAMP. Acetylcholine binding to its receptor raises Ca2+. As illustrated in Figure 8, these intracellular messengers regulate transporters in the ductal cell. The key transporter in the ductal cell is the cystic fibrosis transmembrane conductance regulator (CFTR). CFTR transports both chloride (Cl–) and HCO3–.

Figure 8. The transporters of the pancreatic duct cell.The central purpose of the pancreatic ductal system is to secrete a large volume of water with a high concentration of HCO3–. The pancreatic duct cell is equipped with transporters and mechanisms to accomplish this task. First of all, delivery of large amounts of HCO3– is accomplished by two mechanisms. In one large amounts of carbonic anhyrdrase in ductal cells converts CO2 rapidly to HCO3– and H+. A second mechanism involves a Na+ and HCO3– co-transport on the basolateral surface of the cell. The secretion is mediated by cAMP and Ca2+ intracellular signaling activated by secretin and acetylcholine, respectively. These signals either directly or indirectly have effects on multiple transports that effect the secretory response as described in detail in the text. Adapted from Gorelick F, Pandol, SJ, Topazian M. Pancreatic physiology, pathophysiology, acute and chronic pancreatitis. Gastrointestinal Teaching Project, American Gastroenterological Association. 2003.
Moreover, the Cl– secreted by CFTR exchanges with HCO3– so that the concentration of HCO3– is very high in the secretion. Finally, sodium (Na+) and water follow into the ductal space to counteract the charge and ionic force caused by the HCO3– secretion.
10. What is the importance of pancreatic ductal secretion?
The role of ion and water secretion in maintaining normal exocrine pancreatic function is clear. Loss of function mutations in CFTR as occurs in cystic fibrosis result in severe exocrine pancreatic pathology with fibrosis of the gland associated with loss of exocrine pancreatic tissue. This occurs because the lack of ion and water flow resulting in protein plugging of the pancreatic ducts which leads to inflammation and destruction of functional exocrine tissue. In fact, because patients with cystic fibrosis loose functional exocrine pancreatic tissue, they require life-long treatment with oral preparations of commercially prepared digestive enzymes to digest meals and prevent malnutrition. These observations demonstrate the critical importance of the ion and water secretory pathways in maintenance of normal physiology.
11. How does ingestion of a meal activate the pancreatic secretory response?
Human exocrine pancreatic secretion occurs both during the fasting (interdigestive) state and after ingestion of a meal (digestive). The interdigestive pattern of secretion begins when the upper gastrointestinal tract is cleared of food. In an individual who eats three meals per day, the digestive pattern begins after breakfast and continues until late in the day, after the evening meal is cleared from the upper gastrointestinal tract. This secretion occurs along with a pattern of movements in the intestine that are designed to sweep out material that are remaining in the intestine after the meal keeping the intestine clean to prevent overgrowth of bacteria which can be harmful.
Digestive secretion is divided into 3 phases which are the cephalic, gastric and intestinal phases. The reason for this division is that the network of regulatory systems responsible for effecting secretion shifts as a function of location of the meal and its effect on sensory inputs.
The cephalic phase of the meal represents the time before swallowing of food and considers several stimuli that have inputs during this period. Such stimuli include emotional state, anticipation of the meal, auditory stimuli associated with the meal as well as the smell and taste of the meal and even chewing. The extent of cephalic stimulation of exocrine pancreatic secretion in humans has been evaluated through measurement of exocrine secretions stimulated by sham feeding (chewing and spitting out the food) (8, 9). The input from these sensory stimuli is integrated in the central nervous system nervous system at the dorsal vagal complex and output is transmitted to the exocrine pancreas by the vagus nerve. The cephalic phase can account up to 50% of the pancreatic secretion during a meal. The vagal nerve releases neurotransmitters especially acetylcholine at the pancreatic tissue to cause the secretory responses. Other neurotransmitters released include GRP, substance P and VIP.
The gastric phase of pancreatic secretion results from the effects of the meal in the stomach. The meal in the stomach causes distension which, in turn, activates neural pathways that cause pancreatic secretion (15). When gastric juice and contents of a meal enter the duodenum, a variety of intraluminal stimulants can act on the intestinal mucosa to stimulate pancreatic secretion through both neural and hormonal mechanisms. Three gastric processes—secretion of acid, secretion of pepsin and lipase and emptying—are tightly coupled to the mechanisms of the intestinal phase of pancreatic secretion. Gastric acid entering the duodenum has an effect on bicarbonate secretion from the pancreas. The partial digestion of the protein and carbohydrate of the meal by salivary amylase and gastric pepsin, respectively, create nutrient stimulants that activate pancreatic secretion when delivered to the intestine.
The intestinal phase of pancreatic secretion continues for the duration of the digestive period. It is mediated by both hormones and enteropancreatic vagovagal reflexes. During the intestinal phase the contribution of pancreatic ducts and their secretion of fluid with high concentrations of bicarbonate contribute significantly to the exocrine pancreatic output. The stimulant of this ductal secretion is the acid from the stomach in the duodenum which causes release of secretin from the secretin-containing S cell into the blood (12). The secretin, in turn, interacts with its receptor on the pancreatic ductal cell to cause the fluid and bicarbonate secretion.
Secretion of digestive enzymes during the intestinal phase is mediated by intraluminal fatty acids more than eight carbons in length, monoglycerides of these fatty acids, peptides, amino acids, and, to a small extent, glucose (6, 17, 19-21). The pancreatic enzyme secretory response during the intestinal phase is mediated by both neural and hormonal pathways (Figure 9). Both removing the vagus nerve and administration of the cholinergic antagonist atropine markedly inhibit the digestive enzyme (and bicarbonate) responses to low intestinal loads of amino acids and fatty acids as well as infusion of physiologic concentrations of the hormone cholecystokinin (CCK) (16, 24, 34, 35).

Figure 9. CCK Stimulates Pancreatic Enzyme Secretion by Both Neural and Hormonal Pathways.This cartoon shows the several pathways mediating meal-stimulated pancreatic secretion that involve CCK. First, meal nutrients such as fatty acids, amino acids and peptides delivered from the duodenum stimulate the release of CCK from the CCK-containing I cell to the area around the basolateral surface of the I cell. The CCK released can activate vagal afferent neurons that carry the signal to the dorsal vagal complex where the sensory information is integrated and vagal efferents are activated. Vagal efferents synapse with neurons in the pancreatic ganglia. In turn, via the neurotransmitters, acetylcholine (Ach), gastrin releasing peptide (GRP) and vasoactive intestinal polypeptide (VIP) effector neurons in the pancreatic ganglia activate secretion by pancreatic acinar cells. In addition to activating the neural pathway, CCK released by the I cell enters the general circulation and may act as a hormone on the pancreatic acinar cells to cause secretion. Adapted from Gorelick F, Pandol, SJ, Topazian M. Pancreatic physiology, pathophysiology, acute and chronic pancreatitis. Gastrointestinal Teaching Project, American Gastroenterological Association. 2003.
These results indicate a prominent role for the cholinergic nervous system by activating neural pathways as shown in Figure 9. Further, the results confirm a role for the cholingeric nervous system in bicarbonate secretion stimulated by secretin.
12. Summary
This chapter describes the multiple levels of regulation and coordination that are necessary for normal exocrine pancreatic function and digestion of a meal. The coordination of the regulation of these processes is necessary for meal digestion.
For example, through independent and interacting neurohumoral pathways a meal stimulates secretion of digestive enzymes from pancreatic acini; and ions and water from the pancreatic duct cells into the duodenum. These events require highly developed physiologic responses to the meal resulting in activation and coordination of specialized cellular mechanisms to result in the secretion necessary of meal digestion. This chapter also provides insights into the pathogenesis of pancreatic disorders as they relate to failure of cellular mechanisms responsible for key functions of the exocrine pancreas.
13. References
- Apte MV, Pirola RC and Wilson JS. Battle-scarred pancreas: role of alcohol and pancreatic stellate cells in pancreatic fibrosis. J Gastroenterol Hepatol 21 Suppl 3: S97-S101, 2006. PMID: 16958684.
- Bachem MG, Zhou S, Buck K, Schneiderhan W and Siech M. Pancreatic stellate cells--role in pancreas cancer. Langenbecks Arch Surg 393(6): 891-900, 2008. PMID: 18204855.
- Bachem MG, Zhou Z, Zhou S and Siech M. Role of stellate cells in pancreatic fibrogenesis associated with acute and chronic pancreatitis. J Gastroenterol Hepatol 21 Suppl 3: S92-96, 2006. PMID: 16958683.
- Ballian N and Brunicardi FC. Islet vasculature as a regulator of endocrine pancreas function. World J Surg 31(4): 705-714, 2007. PMID: 17347899.
- Beck IT. The role of pancreatic enzymes in digestion. Am J Clin Nutr 26(3): 311-325, 1973. PMID: 4347665.
- Go VL, Hofmann AF and Summerskill WH. Pancreozymin bioassay in man based on pancreatic enzyme secretion: potency of specific amino acids and other digestive products. J Clin Invest 49(8): 1558-1564, 1970. PMID: 5431665.
- Hofmann AF and Borgstrom B. Hydrolysis of long-chain monoglycerides in micellar solution by pancreatic lipase. Biochim Biophys Acta 70: 317-331, 1963. PMID: 13963970.
- Katschinski M, Dahmen G, Reinshagen M, Beglinger C, Koop H, Nustede R, et al. Cephalic stimulation of gastrointestinal secretory and motor responses in humans. Gastroenterology 103(2): 383-391, 1992. PMID: 1634057.
- Katschinski M, Steinicke C, Reinshagen M, Dahmen G, Beglinger C, Arnold R, et al. Gastrointestinal motor and secretory responses to cholinergic stimulation in humans. Differential modulation by muscarinic and cholecystokinin receptor blockade. Eur J Clin Invest 25(2): 113-122, 1995. PMID: 7737260.
- Kilberg MS, Stevens BR and Novak DA. Recent advances in mammalian amino acid transport. Annu Rev Nutr 13: 137-165, 1993. PMID: 8369142.
- Kimmich GA. Membrane potentials and the mechanism of intestinal Na(+)-dependent sugar transport. J Membr Biol 114(1): 1-27, 1990. PMID: 2181143.
- Konturek SJ, Dubiel J and Gabrys B. Effect of acid infusion into various levels of the intestine on gastric and pancreatic secretion in the cat. Gut 10(9): 749-753, 1969. PMID: 5386632.
- Korc M, Iwamoto Y, Sankaran H, Williams JA and Goldfine ID. Insulin action in pancreatic acini from streptozotocin-treated rats. I. Stimulation of protein synthesis. Am J Physiol 240(1): G56-62, 1981. PMID: 6161541.
- Korc M, Sankaran H, Wong KY, Williams JA and Goldfine ID. Insulin receptors in isolated mouse pancreatic acini. Biochem Biophys Res Commun 84(2): 293-299, 1978. PMID: 363126.
- Kreiss C, Schwizer W, Erlacher U, Borovicka J, Lochner-Kuery C, Muller R, et al. Role of antrum in regulation of pancreaticobiliary secretion in humans. Am J Physiol 270(5 Pt 1): G844-851, 1996. PMID: 8967497.
- Li Y, Hao Y and Owyang C. High-affinity CCK-A receptors on the vagus nerve mediate CCK-stimulated pancreatic secretion in rats. Am J Physiol 273(3 Pt 1): G679-685, 1997. PMID: 9316472.
- Liddle RA, Goldfine ID, Rosen MS, Taplitz RA and Williams JA. Cholecystokinin bioactivity in human plasma. Molecular forms, responses to feeding, and relationship to gallbladder contraction. J Clin Invest 75(4): 1144-1152, 1985. PMID: 2580857.
- Lugea A, Tischler D, Nguyen J, Gong J, Gukovsky I, French SW, et al. Adaptive unfolded protein response attenuates alcohol-induced pancreatic damage. Gastroenterology 140(3): 987-997, 2011. PMID: 21111739.
- Meyer JH and Kelly GA. Canine pancreatic responses to intestinally perfused proteins and protein digests. Am J Physiol 231(3): 682-691, 1976. PMID: 970450.
- Meyer JH, Kelly GA and Jones RS. Canine pancreatic response to intestinally perfused oligopeptides. Am J Physiol 231(3): 678-681, 1976. PMID: 970449.
- Meyer JH, Kelly GA, Spingola LJ and Jones RS. Canine gut receptors mediating pancreatic responses to luminal L-amino acids. Am J Physiol 231(3): 669-677, 1976. PMID: 970448.
- Murphy JA, Criddle DN, Sherwood M, Chvanov M, Mukherjee R, McLaughlin E, et al. Direct activation of cytosolic Ca2+ signaling and enzyme secretion by cholecystokinin in human pancreatic acinar cells. Gastroenterology 135(2): 632-641, 2008. PMID: 18555802.
- Omary MB, Lugea A, Lowe AW and Pandol SJ. The pancreatic stellate cell: a star on the rise in pancreatic diseases. J Clin Invest 117(1): 50-59, 2007. PMID: 17200706.
- Owyang C. Physiological mechanisms of cholecystokinin action on pancreatic secretion. Am J Physiol 271(1 Pt 1): G1-7, 1996. PMID: 8760100.
- Palade G. Intracellular aspects of the process of protein synthesis. Science 189(4200): 347-358, 1975. PMID: 1096303.
- Pandol S, Edderkaoui M, Gukovsky I, Lugea A and Gukovskaya A. Desmoplasia of pancreatic ductal adenocarcinoma. Clin Gastroenterol Hepatol 7(11 Suppl): S44-47, 2009. PMID: 19896098.
- Pandol S, Gukovskaya A, Edderkaoui M, Dawson D, Eibl G and Lugea A. Epidemiology, risk factors, and the promotion of pancreatic cancer: role of the stellate cell. J Gastroenterol Hepatol 27 Suppl 2: 127-134, 2012. PMID: 22320930.
- Pandol SJ, Gorelick FS and Lugea A. Environmental and genetic stressors and the unfolded protein response in exocrine pancreatic function - a hypothesis. Front Physiol 2: 8, 2011. PMID: 21483727.
- Pandol SJ, Saluja AK, Imrie CW and Banks PA. Acute pancreatitis: bench to the bedside. Gastroenterology 132(3): 1127-1151, 2007. PMID: 17383433.
- Petersen OH and Tepikin AV. Polarized calcium signaling in exocrine gland cells. Annu Rev Physiol 70: 273-299, 2008. PMID: 17850212.
- Rinderknecht H. Activation of pancreatic zymogens. Normal activation, premature intrapancreatic activation, protective mechanisms against inappropriate activation. Dig Dis Sci 31(3): 314-321, 1986. PMID: 2936587.
- Sankaran H, Iwamoto Y, Korc M, Williams JA and Goldfine ID. Insulin action in pancreatic acini from streptozotocin-treated rats. II. Binding of 125I-insulin to receptors. Am J Physiol 240(1): G63-68, 1981. PMID: 7006418.
- Scheele G, Bartelt D and Bieger W. Characterization of human exocrine pancreatic proteins by two-dimensional isoelectric focusing/sodium dodecyl sulfate gel electrophoresis. Gastroenterology 80(3): 461-473, 1981. PMID: 6969677.
- Singer MV, Niebel W, Jansen JB, Hoffmeister D, Gotthold S, Goebell H, et al. Pancreatic secretory response to intravenous caerulein and intraduodenal tryptophan studies: before and after stepwise removal of the extrinsic nerves of the pancreas in dogs. Gastroenterology 96(3): 925-934, 1989. PMID: 2914653.
- Singer MV, Solomon TE and Grossman MI. Effect of atropine on secretion from intact and transplanted pancreas in dog. Am J Physiol 238(1): G18-22, 1980. PMID: 6986796.
- Vonlaufen A, Phillips PA, Xu Z, Goldstein D, Pirola RC, Wilson JS, et al. Pancreatic stellate cells and pancreatic cancer cells: an unholy alliance. Cancer Res 68(19): 7707-7710, 2008. PMID: 18829522.
- Weiss FU. Pancreatic cancer risk in hereditary pancreatitis. Front Physiol 5: 70, 2014. PMID: 24600409.
- Whitcomb DC, Gorry MC, Preston RA, Furey W, Sossenheimer MJ, Ulrich CD, et al. Hereditary pancreatitis is caused by a mutation in the cationic trypsinogen gene. Nat Genet 14: 141-145, 1996. PMID: 8841182.
- Whitcomb DC and Lowe ME. Human pancreatic digestive enzymes. Dig Dis Sci 52(1): 1-17, 2007. PMID: 17205399.
- Williams JA. Intracellular signaling mechanisms activated by cholecystokinin-regulating synthesis and secretion of digestive enzymes in pancreatic acinar cells. Annu Rev Physiol 63: 77-97, 2001. PMID: 11181949.
- Wright EM, Martin MG and Turk E. Intestinal absorption in health and disease--sugars. Best Pract Res Clin Gastroenterol 17(6): 943-956, 2003. PMID: 14642859.
- Yadav D and Lowenfels AB. The epidemiology of pancreatitis and pancreatic cancer. Gastroenterology 144(6): 1252-1261, 2013. PMID: 23622135.