Entry Version:
Citation:
Pancreapedia: Exocrine Pancreas Knowledge Base, DOI: 10.3998/panc.2012.10
Attachment | Size |
---|---|
![]() | 385.53 KB |
Gene Symbol: Insr
1. General Information
The existence of a specific membrane receptor for insulin had been postulated earlier with the showing that insulin stimulated glucose uptake into cells but the current era began with the showing in 1971 that radioiodinated insulin bound to specific saturable sites on fat cells and their membranes (10). This led to numerous measurements of the affinity and capacity of the binding sites for insulin (IR) on various cells and isolated membranes. One of the early complications was the finding of curvilinear Scatchard Plots for insulin binding and other evidence of negative cooperativity. Following the crosslinking of radiolabeled insulin to the receptor the subunit structure of the IR was elucidated leading to a model of two extracellular α subunits of 135 kDa and two transmembrane β subunits of 95 kDa linked by disulfide bonds (33,44). These subunits were found to derive from a 220 kDa precursor which was synthesized in RER and transported to the Golgi where it was dimerized, cleaved into α and β subunits, glycosylated and transported to the plasma membrane in its correct orientation.
In 1982, it was noted that the receptor was associated with tyrosine kinase activity (40) and then that the β subunit was itself a tyrosine kinase (22) that could phosphorylate both itself and external proteins. The sites for autophosphorylation were being identified when the clonining of the IR in 1985 by the groups of Axel Ulrich at Genentech (52) and Bill Rutter at UCSF (13) accelerated the process. The gene was cloned in 1989 (45) and has 22 exons. Exon 11, the terminal end of the eventual α subunit is alternatively spliced leading to IR-A and IR-B isoforms; IGF-I binds to IR-A with one hundred times lower affinity than insulin. The modular structure of the insulin receptor is shown in Fig. 1. The α subunit contains two leucine rich regions (L1 and L2), a cysteine rich region and two fibronectin like domains. The β subunit contains a extracellular fibronectin domain, a transmembrane domain, a juxtamembrane domain, a tyrosine kinase domain and a C-terminal domain. For further information consult the following reviews (12,31,51).
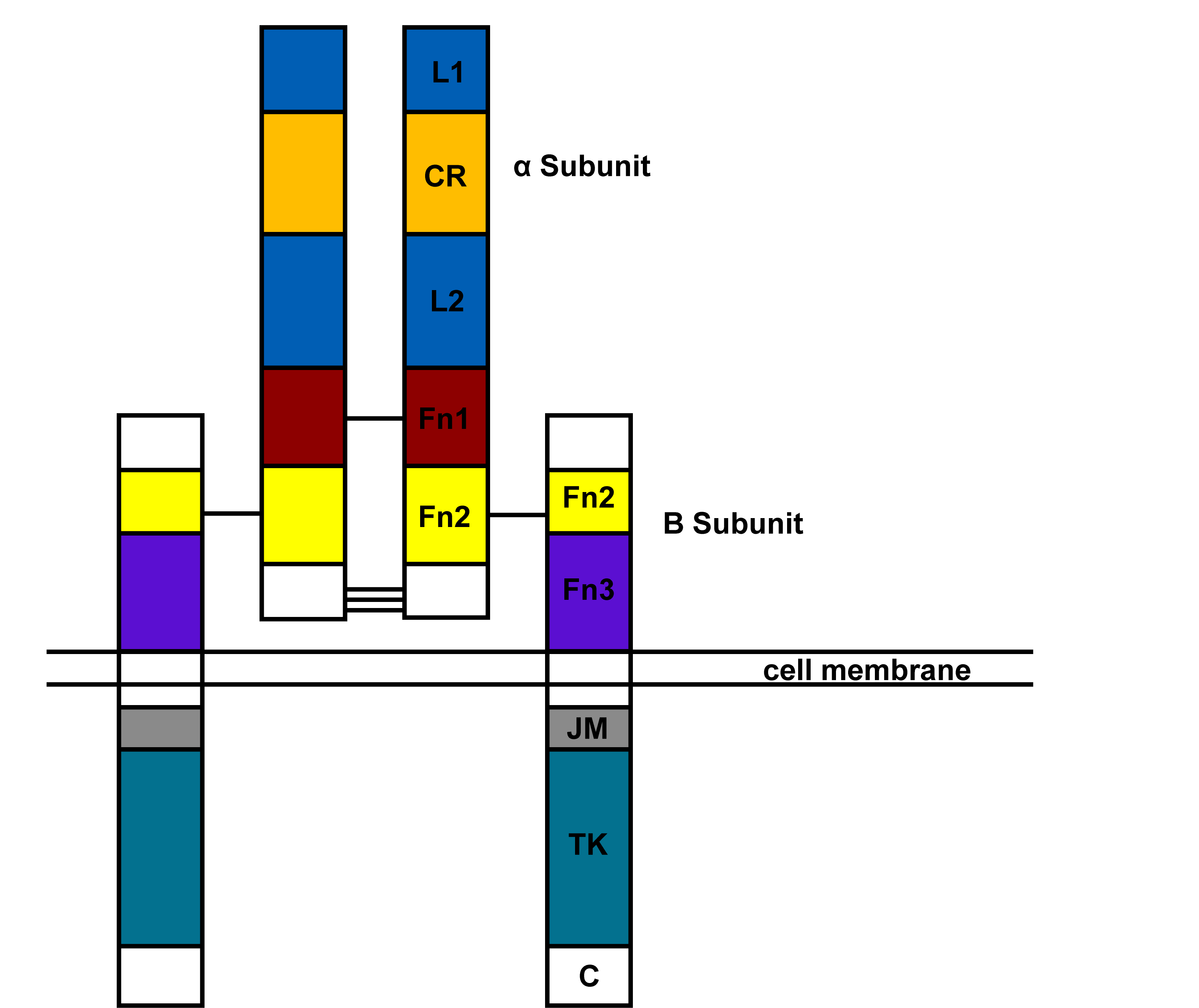
Figure 1. Domain structure of insulin receptor based on primary sequence.
In 2006, the IR-A ectodomain dimer was crystallized (34). The structure revealed a folded-over conformation that places the ligand binding regions of the two chains in juxtaposition. Binding domains are in the L1 and first fibronectin repeat. Each receptor binds two insulin molecules each of which binds two different regions one with high and the other with lower affinity (11,50). This model can explain the negative cooperativity of binding. The IGF-I receptor is also believed to have a similar structure (49).
Concurrent with understanding of the IR ectodomain structure and insulin binding, there has been ongoing progress in understanding the signaling mechanism of the intracellular β subunit. The kinase activity of the receptor phosphorylates a number of tyrosines on the receptor which serve as docking sites and also phosphorylates intracellular substrates. Most, if not all, of insulin’s biological effects are mediated by the insulin receptor tyrosine kinase (51). The most important of these substrates is IRS-1 (Insulin Receptor Substrate 1) to which SH2 domain proteins including PI3-Kinase, SH-PTP2, Grb2, and Nck bind and signal downstream. Through these molecules the IR activates Akt, the mTOR pathway, and the ERK pathway. Negative feedback regulation of IRS-1 occurs by serine phosphorylation. Other substrates of the IR kinase include other IRS proteins, Gab2, Shc, and Cbl, the latter of which plays a role in stimulating movement of GLUT4 containing vesicles to the plasma membrane in muscle and fat cells. For detailed coverage see the following reviews (2,23,48,51).
More integrative studies of IR signaling and action have come from gene deletion studies (24). Mice lacking IR throughout the body are born normally but die of ketoacidosis in the newborn state. Mice lacking IR in specific target cells through targeted deletion have provided insight into the metabolic functions of specific organs and uncovered a role for IR signaling in the brain and pancreatic beta cells, as well as the classic metabolic target tissues, fat, liver, and muscle.
2. Insulin Receptors in The Exocrine Pancreas
Insulin is known to have multiple actions on the exocrine pancreas including maintaining the ability to secrete digestive enzymes (53), regulating amylase gene expression (26), and maintaining the size of the pancreas and its ability to regenerate after pancreatitis (20). Diabetes in children can be associated with pancreatic insufficiency (15,29). Animal studies of the effect of diabetes on the exocrine pancreas have primarily been carried out using the beta cell toxin streptozotocin. In vitro effects of insulin on rodent acinar cells include stimulation of glucose uptake (19,55), protein synthesis (25,30), potentiation of CCK-induced secretion (1,41), and in pancreas derived AR42J cells, stimulation of mitogenesis (37). At the signal transduction level insulin activates Akt phosphorylation and mTOR signaling (47).
Insulin receptors on pancreatic acinar cells were first demonstrated by the binding of 125I-insulin in a saturable manner to isolated acini from mouse, rat and guinea pig (27,43,45). Scatchard analysis of binding were biphasic and showed high affinity sites with a Kd of about 1.5 nM and capacity of about 10,000 receptors per cell; low affinity sites were much more numerous with a Kd of 88 nM for mouse and 998 nM for rat. Addition of unlabeled insulin accelerated dissociation from rat acini consistent with negative cooperativity rather than two distinct sites (43). The receptor bound insulin analogs with varying potency (insulin > desdipeptide insulin > proinsulin > desoctapeptide insulin) that was similar to the ability of the analogs to increase protein synthesis in acini from diabetic rats (25). Guinea pig insulin is significantly different from other species and guinea pig acinar receptors bound bovine insulin with a higher affinity than guinea pig insulin or porcine proinsulin (46). Mouse acini were also shown to possess distinct receptors for IGF-I and IGF-II (54).
The localization of insulin binding to acinar and other cells in the pancreas has been demonstrated by light and EM autoradiography. When 125I-Insulin was injected intravenously, saturable uptake was observed by the pancreas (3,9,42). EM autoradiographs showed silver grains localized both over the plasma membrane and intracellularly in acinar cells. Saturable binding was also observed over duct cells (42). In an in vitro study of 125I-Insulin binding to rat acini, silver grains from 125I were primarily over the plasma membrane at 3 min but by 30 min were primarily intracellular (16). Studies in other cell types have shown that both insulin and its receptor are internalized although the function of internalized insulin is unclear.
Insulin receptors on acinar cells are also subject to regulation. Insulin down regulates its own receptor in a variety of cells and acinar cells are exposed to high concentrations of insulin through the islet-acinar portal blood system (1,53). Acini prepared from diabetic mice showed an increased number of insulin receptors compared to normal mice and addition of insulin to 24 hour cultured acini decreased insulin binding (36). Using pancreatic AR42J cells, insulin decreased the biosynthesis of insulin receptors in part by decreasing IR mRNA levels (37,38). The binding of insulin was also affected by CCK and other secretagogues (39). CCK, carbamylcholine, active phorbol ester and calcium ionophore (A23187) all decreased high affinity binding without affecting insulin internalization of degradation.
The occupancy of insulin receptors has also been related to the biological effects of insulin on acinar cells. Insulin increased glucose transport (uptake) by acini from normal and diabetic mice (27,55) with a greater effect on acini from diabetic animals that was seen at lower concentrations of insulin. This greater effect is due both to lower contaminating insulin levels and IR upregulation. In acini from diabetic rats and mice insulin increased protein synthesis as shown by incorporation of radioactive amino acids (25,55). Of likely relevance to protein synthesis, insulin rapidly increased the phosphorylation of ribosomal protein S6 (7,47). The concentration dependence and analog specificity of these actions of insulin is similar to the data for receptor occupancy (17).
More recently IR have been demonstrated in pancreatic acini by immunoblotting of the beta subunit of the receptor (Sans, Vogel and Williams, unpublished data). The subunit had a Mr of about 95 kDa and was essentially absent after deletion of the IR gene in acinar cells. The biological response to insulin in these mice was greatly reduced.
3. Tools to Study Insulin Receptors
a. cDNA clones
cDNA clones for the insulin receptor are commercially available at OriGene, GeneCopoeia, DNASU Plasmid Repository.
The specific mutation Q1152 next to the key tyrosine autophosphorylation sites (Y1146, Y1150, Y1151) of the insulin receptor deregulates insulin receptor kinase and generates insulin insensitivity in cells (14). Other dominant-negative mutations in the insulin receptor have been described (8,32).
b. Antibodies
Antibodies raised to different epitopes of the α and ß subunits of the human insulin receptor are commercially available through Santa Cruz Biotechnology, Inc. The Insulin Rß (C-19) (sc-711) is an affinity purified rabbit antibody raised against a peptide mapping at the C-terminus of Insulin Rß of human origin, that detects Insulin Rß of mouse, rat, and human origin by WB, immunoprecipitation, immunoflorescence and solid phase ELISA. This antibody works well in our laboratory to detect the IRß by WB of mouse pancreas samples. Antibodies for the insulin receptor are also commercially available at companies, such as Cell Signaling, Abcam, Novus Biologicals, My Biosource, but we have not tested them in our lab.
c. Transgenic Mice
The generation of mice bearing Insr mutations has been instrumental to dissecting the pathogenesis of insulin resistance, diabetes, and growth retardation, as well as to identify new elements in insulin signaling and assign roles to known insulin receptor substrates. Mice homozygous for null insulin receptor alleles are born at term with slight growth retardation (about 10%), but without apparent metabolic abnormalities. After birth, metabolic control rapidly deteriorates and mice die of ketoacidosis (24). The phenotype of Insr heterozygous knockouts has also been studied, since it is relevant to genetic predisposition to diabetes in humans with mutations in the INSR.
In contrast, mice lacking insulin receptors in specific cell types as a result of conditional mutagenesis develop mild metabolic and reproductive abnormalities, and allow for detailed studies of insulin receptor function in different tissues in adult mice. Most of these experiments require a binary system in which floxed Insr is bred to a tissue specific Cre-producing strain of mice. By using this Cre/loxP system the following conditional tissue specific conditional knockout mice have been generated and studied:
MIRKO. The conditional muscle insulin receptor knockout mouse has impaired insulin signaling and decreased insulin-dependent glucose transport, without systemic insulin resistance, but develops metabolic syndrome and dyslipidemia (5).
FIRKO. The conditional white and brown fat knockout have been created using a Cre transgene driven by the adipose-specific aP2 promoter, and show decreased fat mass and resistance to gain weight during aging (4).
BATIRKO. Insr has been inactivated in the brown adipose tissue alone, using the uncoupling protein-1 (UCP-1) promoter to drive Cre expression. These mice have age-dependent loss of brown adipose tissue with decreased ß-cell function (8).
LIRKO. The liver-specific conditional insulin receptor knockout mice were generated using the Cre/lox system with an albumin promoter to drive Cre expression in the liver only. These mice show insulin resistance, glucose intolerance, with hyperinsulinemia (35).
ßIRKO. The Insr has also been inactivated in mature pancreatic ß–cells of mice, using insulin/Cre-dependent recombination; these mice show a selective impairement of glucose-dependent insulin release, and age-dependent glucose intolerance (28).
NIRKO. The conditional neuron specific Insr knockout mice have been generated using nestin/Cre-mediated ablation. These mice have increased food intake, moderate diet-dependent obesity, and hypogonadotropic hypogonadism, with impaired maturation of ovarian follicles in females and reduced spermatogenesis in males (6).
PACIRKO. Conditional pancreas acinar cell specific Insr knockout mice have been generated in our laboratory using the elastase-driven Cre/lox-dependent system. The Insr/lox mice were generated by Dr. Ron Kahn (35) and the elastase-Cre mice by Drs. Baoan Ji and Craig Logsdon (21). The PACIRKO mice are being currently characterized and used to study the role of insulin in pancreatic acinar cells.
Kinase-deficient human insulin receptor overexpression in muscle of transgenic mice (8). In these mice, the overexpression of a kinase-deficient human insulin receptor causes dominant-negative effects at the level of receptor kinase activation, decreased insulin sensitivity in vivo and can be used to create novel animal models of tissue-specific insulin resistance.
4. References
- Barreto SG, Carati CJ, Toouli J, Saccone GTP. The islet-acinar axis of the pancreas: more than just insulin. Am J Physiol Gastrointest Liver Physiol. 299: G10-G22, 2010. PMID 20395539
- Belfior A, Frasca F, Pandini G, Sciacca L, Vigneri R. Insulin receptor isoforms and insulin receptor/insulin-like growth factor receptor hybrids in physiology and disease. Endocr Rev., 30: 586-623, 2009. PMID: 19752219
- Bergeron JJM, Rachubinski R, Searle N, Sikstrom R, Borts D, Bastian P, Posner BI. Radioautographic visualization of in vivo insulin binding to the exocrine pancreas. Endocrinology, 107: 1069-1080, 1980. PMID: 6997018
- Blüher M, Michael MD, Peroni OD, Ueki K, Carter N, Kahn BB, Kahn CR. Adipose tissue selective insulin receptor knockout protects against obesity and obesity-related glucose intolerance. Dev Cell., 3:25-38, 2002. PMID 12110165
- Brüning JC, Michael MD, Winnay JN, Hayashi T, Hörsch D, Accili D, Goodyear LJ, Kahn CR. A muscle-specific insulin receptor knockout exhibits features of the metabolic syndrome of NIDDM without altering glucose tolerance. Mol Cell. , 2:559-69, 1998. PMID 9844629
- Brüning JC, Gautam D, Burks DJ, Gillette J, Schubert M, Orban PC, Klein R, Krone W, Müller-Wieland D, Kahn CR. Role of brain insulin receptor in control of body weight and reproduction. Science., 289:2122-5, 2000. PMID 11000114
- Burnham DB and Williams JA. Effects of carbachol, cholecystokinin, and insulin on protein phosphorylation in isolated pancreatic acini. J. Biol Chem., 257:10523-10528. PMID 7050109
- Chang PY, Benecke H, Le Marchand-Brustel Y, Lawitts J, Moller DE. Expression of a dominant-negative mutant human insulin receptor in the muscle of transgenic mice. J Biol Chem., 269:16034-40, 1994. PMID 8206901
- Cruz J, Posner BI, Bergeron JJM. Receptor-mediated endocytosis of [125I]insulin into pancreatic acinar cells in vivo. Endocrinology, 115: 1996-2008, 1984. PMID: 6386447
- Cuatrecasas P. Insulin--receptor interactions in adipose tissue cells: direct measurement and properties. Proc Natl Acad Sci USA., 68: 1264-1268, 1971. PMID: 5288373
- De Meyts P. The insulin receptor: a prototype for dimeric, allosteric membrane receptors? Trends Biochem Sci., 33: 376-384, 2008. PMID 18640841
- De Meyts P. Insulin and its receptor: structure, function and evolution. Bioessays., 26: 1351-1362, 2004. PMID: 15551269
- Ebina Y, Ellis L, Jarnagin K, Edery M, Graf L, Clauser E, Ou JH, Masiarz F, Kan YW, Goldfine ID, et al. The human insulin receptor cDNA: the structural basis for hormone-activated transmembrane signalling. Cell, 40: 747-758, 1985. PMID 2859121
- Formisano P, Sohn KJ, Miele C, Di Finizio B, Petruzziello A, Riccardi G, Beguinot L, Beguinot F. Mutation in a conserved motif next to the insulin receptor key autophosphorylation sites de-regulates kinase activity and impairs insulin action. J Biol Chem., 268:5241-8, 1993. PMID 8383132
- Frier BM, Saunders JH, Wormsley KG, Bouchier IA. Exocrine pancreatic function in juvenile-onset diabetes mellitus. Gut. 17:685-91, 1976. PMID 976808
- Goldfine ID, Kriz BM, Wong KY, Hradek G, Jones AL, Williams JA. Insulin action in pancreatic acini from streptozotocin-treated rats. III. Electron microscope autoradiography of 125I-insuln. Am j Physiol., 240: G69-G75, 1981. PMID 7006419
- Goldfine ID, Williams JA. Receptors for insulin and CCK in the acinar pancreas: relationship to hormone action. Int Rev Cytol., 85: 1-38, 1983. PMID 6198304
- Guerra C, Navarro P, Valverde AM, Arribas M, Brüning J, Kozak LP, Kahn CR, Benito M. Brown adipose tissue-specific insulin receptor knockout shows diabetic phenotype without insulin resistance. J Clin Invest. 108:1205-13, 2001. PMID 11602628
- Han J, Liu YQ. Suppressed glucose metabolism in acinar cells might contribute to the development of exocrine pancreatic insufficiency in streptozotocin-induced diabetic mice. Metabolism. 59:1257-67, 2010. PMID 20051281
- Hegyi P, Rakonczay-Jr Z, Sari R, Czako L, Farkas N, Gog C, Nemeth J, Lonovics J, Takacs T. Insulin is necessary for the hypertrophic effect of cholecystokinin-octapeptide following acute necrotizing experimental pancreatitis. World J Gastroenterol. 10:2275-7, 2004. PMID 15259081
- Ji B, Song J, Tsou L, Bi Y, Gaiser S, Mortensen R, Logsdon CD. Robust acinar cell transgene expression of CreErT via BAC recombineering. Genesis, 68:918-26, 2008. PMID 18693271
- Kasuga M, Karlsson FA, Kahn CR. Insulin stimulates the phosphorylation of the 95,000-dalton subunit of its own receptor. Science, 215: 185-187, 1982. PMID 7031900
- Kido Y, Nakae J, Accili D. Clinical review 125: The insulin receptor and its cellular targets. J Clin Endocrinol Metab., 86: 972-979, 2001. PMID 11238471
- Kitamura T, Kahn CR, Accili D. Insulin receptor knockout mice. Annu Rev Physiol., 65: 313-332, 2003. PMID 12471165
- Korc M, Iwamoto Y, Sankaran H, Williams JA, Goldfine ID. Insulin action in pancreatic acini from streptozotocin-treated rats I. Stimulation of protein synthesis. Am J Physiol, 240: G56-G62, 1981. PMID 6161541
- Korc M, Owerbach D, Quinto C, Rutter WJ. Pancreatic islet-acinar cell interaction: amylase messenger RNA levels are determined by insulin. Science 213:351-353, 1981. PMID 6166044
- Korc M, Sankaran H, Wong KY, Williams JA, Goldfine ID. Insulin receptors in isolated mouse pancreatic acini. Biochem Biophys Res Commun., 84: 293-299, 1978. PMID 363126
- Kulkarni RN, Brüning JC, Winnay JN, Postic C, Magnuson MA, Kahn CR. Tissue-specific knockout of the insulin receptor in pancreatic beta cells creates an insulin secretory defect similar to that in type 2 diabetes. Cell., 96:329-39, 1999. PMID 10025399
- Laass MW, Henker J, Thamm K, Neumeister V, Kuhlisch E. Exocrine pancreatic insufficiency and its consequences on physical development and metabolism in children and adolescents with type 1 diabetes mellitus. Eur J Pediatr., 163:681-2, 2004. PMID 15300430
- Lahaie RG. Translational control of protein synthesis in isolated pancreatic acini: role of CCK8, carbachol, and insulin. Pancreas., 1:403-10, 1986. PMID 2436215
- Lee J, Pilch PF. The insulin receptor: structure, function, and signaling. Am J Physiol., 266: C319-C334, 1994. PMID 8141246
- Levy-Toledano R, Caro LH, Accili D, Taylor SI. Investigation of the mechanism of the dominant negative effect of mutations in the tyrosine kinase domain of the insulin receptor. EMBO J. 13:835-42, 1994. PMID 8112298
- Massagué J, Czech MP. Role of disulfides in the subunit structure of the insulin receptor. Reduction of class I disulfides does not impair transmembrane signalling. J Biol Chem., 257: 6729-6738, 1982. PMID 7045094
- McKern NM, Lawrence MC, Streltsov VA, Lou MZ, Adams TE, Lovrecz GO, Elleman TC, Richards KM, Bentley JD, Pilling PA, Hoyne PA, Cartledge KA, Pham TM, Lewis JL, Sankovich SE, Stoichevska V, Da Silva E, Robinson CP, Frenkel MJ, Sparrow LG, Fernely RT, Epa VC, Ward CW. Structure of the insulin receptor ectodomain reveals a folded-over conformation. Nature, 443: 218-221, 2006. PMID 16957736
- Michael MD, Kulkarni RN, Postic C, Previs SF, Shulman GI, Magnuson MA, Kahn CR. Loss of insulin signaling in hepatocytes leads to severe insulin resistance and progressive hepatic dysfunction. Mol Cell., 6:87-97, 2000. PMID 10949030
- Mössner J, Logsdon CD, Goldfine ID, Williams JA. Regulation of pancreatic acinar cell insulin receptor by insulin. Am J Physiol., 247: G155-G160, 1984. PMID 6380310
- Mössner J, Logsdon CD, Williams JA, Goldfine ID. Insulin, via its own receptor, regulates growth and amylase synthesis in pancreatic acinar AR42J cells. Diabetes, 34:891-7, 1985. PMID 2411617
- Okabayashi Y, Maddux BA, McDonald AR, Logsdon CD, Williams JA, Goldfine ID. Mechanisms of insulin-induced insulin-receptor downregulation. Decrease of receptor biosynthesis and mRNA levels. Diabetes, 38: 182-187, 1989. PMID 2644141
- Okabayashi Y, Otsuki M, Nakamura T, Koide M, Hasegawa H, Okutani T, Kido Y. Regulatory effect of cholecystokinin on subsequent insulin binding to pancreatic acini. Am J Physiol., 258: E562-E568, 1990. PMID 2110419
- Petruzzelli LM, Ganguly S, Smith CJ, Cobb MH, Rubin CS, Rosen OM. Insulin activates a tyrosine-specific protein kinase in extracts of 3T3-L1 adipocytes and human placenta. Proc Natl Acad Sci USA., 79: 6792-6796, 1982. PMID 6294652
- Saito A, Williams JA, Kanno T. Potentiation of cholecystokinin-induced exocrine secretion by both exogenous and endogenous insulin in isolated and perfused rat pancreata. J Clin Invest. 65:777-782, 1980. PMID 6987265
- Sakamoto C, Williams JA, Roach E, Goldfine ID. In vivo localization of insulin binding to cells of the rat pancreas. Proc Soc Exp Biol and Medicine, 175: 497-502, 1984. PMID 2982685
- Sankaran H, Iwamoto Y, Korc M, Williams JA, Goldfine ID. Insulin action in pancreatic acini from streptozotocin-treated rats. II. Binding of 125I-insulin to receptors. Am J Physiol., 240: G63-G68, 1981. PMID 7006418
- Siegel TW, Ganguly S, Jacobs S, Rosen OM, Rubin CS. Purification and properties of the human placental insulin receptor. J Biol Chem., 256: 9266-9273, 1981. PMID 7021559
- Seino S, Seino M, Nishi S, Bell GI. Structure of the human insulin receptor gene and characterization of its promoter. Proc Natl Acad Sci USA., 86: 114-118, 1989. PMID 2911561
- Sjödin L, Holmberg K, Lyden A. Insulin receptors on pancreaptic acinar cells in guinea pigs. Endocrinology, 115: 1102-1109, 1984. PMID 6086285
- Sung CK, Williams JA. Insulin and ribosomal S6 kinase in rat pancreatic acini. Diabetes, 38: 544-549, 1989. PMID 2653925
- Taniguchi CM, Emanuelli B, Kahn CR. Critical nodes in signalling pathways: insights into insulin action. Nat Rev Mol Cell Biol., 7: 85-96, 2006. PMID 16493415
- Ward CW, Lawrence MC, Streltsov VA, Adams TE, McKern NM. The insulin and EGF receptor structures: new insights into ligand-induced receptor activation. Trends Biochem Sci., 32: 129-137, 2007. PMID 17280834
- Ward C, Lawrence M, Streltsov V, Garrett T, McKern N, Lou MZ, Lovrecz G, Adams T. Structural insights into ligand-induced activation of the insulin receptor. Acta Physiol (Oxf)., 192: 3-9, 2008. PMID 18171424
- White MF, Kahn CR. The insulin signaling system. J Biol Chem., 269: 1-4, 1994. PMID 8276779
- Ullrich A, Bell JR, Chen EY, Herrera R, Petruzzelli LM, Dull TJ, Gray A, Coussens L, Liao YC, Tsubokawa M, et al. Human insulin receptor and its relationship to the tyrosine kinase family of oncogenes. Nature, 313: 756-761, 1985. PMID 2983222
- Williams JA, Goldfine ID. The insulin-acinar relationship. In The Pancreas: Biology, Pathobiology and Disease. Second Edition ed by VLW Go. Raven Press, New York pp 789-802, 1993.
- Williams JA, Bailey A, Humbel R, Goldfine ID. Insulinlike growth factors bind to specific receptors in isolated pancreatic acini. Am J Physiol., 246: G96-G99, 1984. PMID 6320665
- Williams JA, Bailey AC, Preissler M, Goldfine ID. Insulin regulation of sugar transport in isolated pancreatic acini from diabetic mice. Diabetes, 31: 674-682, 1982. PMID 6761207