Entry Version:
Citation:
Pancreapedia: Exocrine Pancreas Knowledge Base, DOI: 10.3998/panc.2017.07
Attachment | Size |
---|---|
![]() | 510.49 KB |
![]() | 235.14 KB |
Gene Symbols: ITPR1, ITPR2, ITPR3
Other Names: IP3R1, IP3R2, IP3R3
1. General: The role of InsP3R in pancreatic acinar and other cells
The classical pathway linking stimulation by neurotransmitters and hormones to changes in phosphoinositide metabolism and the subsequent InsP3 induced Ca2+ release was in large part documented by work carried out in exocrine cells, in particular, acinar cells isolated from the exocrine pancreas (62). In seminal work using permeabilized rat pancreatic acini, Streb and colleagues demonstrated that the addition of InsP3 resulted in Ca2+ release from a non-mitochondrial Ca2+ store (54). This store was later identified to be the endoplasmic reticulum (ER) through experiments where sub-cellular fractions from pancreatic cells were isolated and exposed to InsP3 (6, 53). Ca2+ release is rapid, occurring as quickly as 1s following secretagogue stimulation of acinar cells. Ca2+ release is also isomer specific, with other structurally related inositol phosphates shown to be far less efficacious in releasing Ca2+(54). The target protein for InsP3 binding was later identified as the inositol 1,4,5-trisphosphate receptor (InsP3R) (56). Strikingly, the secretion of digestive enzymes from pancreatic acinar cells has been shown to be entirely dependent on the activation of InsP3Rs and the resulting elevation in intracellular [Ca2+] (22). The receptor is encoded by 3 distinct genes in mammalian cells (ITPR1, ITPR2, ITPR3) that generate 3 monomeric isoforms (R1, R2, R3 respectively) that share 60-70% sequence homology (47).
Originally purified and cloned from rat cerebellum, the full length InsP3R forms a tetrameric cation selective channel in vivo (40). The three isoforms of InsP3R exhibit overlapping patterns of expression, with most cells and tissues expressing more than one isoform (63). Western blotting and quantitative PCR has revealed that there is relatively equal expression of the R2 and R3 isoforms in pancreas, with R1 only constituting ~3% of the total InsP3R (63). The importance ofR2 and R3 isoforms to pancreatic Ca2+ signaling is most evident in studies employing InsP3R knockout (KO) mice (22). Although single R2 or R3 KO mice were found to have no observable ill effects or phenotypic alterations, double R2/R3 KO mice typically gained less body weight post-natally compared to single KO or WT mice. Furthermore, double KO mice tended to lose weight rapidly post weaning and typically died 4 weeks after birth. These observations were attributed to the key role the R2 and R3 isoforms play in the secretion of saliva and exocytosis of digestive enzymes from exocrine glands and the resulting inability of double KO mice to swallow or properly digest dry adult food. Indeed, dou ble KO mice were rescued and reached body weights comparable to WT when a fed pre-digested wet mash diet or an elemental diet. Pancreatic acinar cells from R2/R3 double KO mice were also found to accumulate zymogen granules and failed to secrete zymogen granules on stimulation with secretagogues. The study also highlights the inability of R1 to rescue double R2/R3 KO mice (22).
The fidelity and specificity of the Ca2+ signal required for exocytosis is thought to be largely determined by the differential expression, localization and modulation exhibited by the 3 InsP3R isoforms (21). For the most part , InsP3Rs are predominantly localized to the ER, although the golgi, nucleus, plasma membrane, peroxisomes and endolysosomal vesicles have also been reported to express small levels of InsP3Rs. Pancreatic acinar cells are highly polarized, both functionally and morphologically and InsP3Rs are predominantly expressed in ER extensions in the apical regions juxtaposed to the acinar lumen (Figure 1) (34, 36, 42, 69). Using fluorescence imaging techniques, studies have shown that stimulating InsP3 generation in these cells results in the initiation of Ca2+ signals in the apical regions, followed by the propogation basally of a Ca2+ wave that is facilitated by peripheral InsP3Rs. Stimulation of basal secretagogue receptors as well as uncaging InsP3 in various regions of the cell also confirm that Ca2+ signals initiate in apical regions of the pancreatic acinar cell (5, 20, 31, 58, 65).
Figure 1: Localization of InsP3Rs in pancreatic acinar cells. Immunofluorescence localizations in pancreatic lobules of InsP3Rs by confocal microscopy demonstrate the localization of InsP3R1, R2, R3 and amylase (A,B,C,D respectively) respectively. All 3 isoforms are predominantly localized to the apical pole of acinar cells directly abutting the plasma membrane (arrows in panels A-C). R1 and R3 also appeared to localize to perinuclear structures. (Scale bar = 10 μm). (69)
2. Structural features of the InsP3R
Based on the sequence homology, all three isoforms of InsP3R are predicted to have the same general structural domains (12). Specifically, the InsP3R monomer is conventionally divided into 3 functional domains: an N-terminal ligand binding domain (LBD), a coupling and modulatory domain and a C-terminal transmembrane domain (TMD) that contains the channel (21) (Figure 2A). To date, the majority of structural insights were from the investigations of R1, including the recent structures of R1 revealed by cryo-EM (Figure 2B and 2C) andx-ray crystallography (26).Therefore, in this review, the locations of common functional modules in InsP3R, which are also present in R2 and R3 at homologous regions, are described using the amino acid sequence of R1. InsP3 binding is mediated by the ‘core’ ligand binding domain, which constitutes amino acids (AA) 224-578 of the LBD. This region contains 10 conserved positively charged arginine and lysine residues (3 critical, R265, K508, R511) that are thought to allosterically coordinate the negatively charged PO43- groups of InsP3 in a binding pocket (67). By using concatenated subunits (1, 18), our group has recently reported that InsP3 molecules must be bound to each monomer of the four subunits to open the channel and activate InsP3R (2). This gating mechanism ensures the fidelity of InsP3 induced calcium release and may provide mechanistic insight into diseases where mutations have an impact on InsP3 binding to the receptor. The three isoforms have differing InsP3 binding affinities that are regulated by the first 223 AA of the LBD, termed the suppressor domain (SD). Specifically, competitive InsP3 binding assays using GST fusion constructs encoding AA 1-604 demonstrate that R2 has an 11-fold greater affinity (Kd) for InsP3 compared to R3 (14 nM vs. 163 nM), with R1 having an intermediate affinity (49.5 nM) (29). Similar studies in pancreatic acini revealed a Kd of 6 nM (24), comparable to that of R2, while permeabilized Ca2+ release assays in acinar cells demonstrated an EC50 for InsP3 of 0.8 µM (45) (Figure 3).Deletion of the suppressor domain (SD) results in the loss of distinct InsP3 affinities between the isoforms and a 10-100 fold increase in InsP3 affinity (29). Despite an increased InsP3 binding affinity, deletion of the SD also results in the loss of channel activity, indicating that the SD is required for inducing InsP3R activation and Ca2+ release. The pivotal role of SD for InsP3R activation was also strongly supported by Cryo-EM structure of homotetrameric R1 solved at near-atomic resolution (19). The Serysheva group showed that the SD domain forms inter-subunit interactions with ARM2, ARM3, β-TF2 and CTD domains of adjacent subunits, which may underlie a direct functional coupling between the N- and C- terminus of the receptor (19). Based on hydropathy plots, the TMD is similar in structure to that of RyRs and voltage gated K+, Na+ and Ca2+ channels and constitutes 6 putative transmembrane regions (TM1-6) (16). The TMD is responsible for the ER targeting (44, 46) and for the oligomerization of the InsP3R into tetramers, which occurs co-translationally (30). Lastly, TM5 and 6 forms the pore through which Ca2+ is conducted (48). The loop between TM5 and 6 contains a selectivity filter (GVGD; similar to the super family of cation selective channels) that provides some degree of cation selectivity to the InsP3R (21). However, it is poorly Ca2+ selective and allows conduction of monovalent cations (Ca2+:K+ = 6:1). In fact, it is believed that “functional” Ca2+ selectivity of InsP3R is primarily determined by virtue of SERCA being a highly selective Ca2+ pump and Ca2+ being by the far the most abundant cation in the ER. To “gate” and open the channel, . The CT tail (last 160 AA) and the large (1700 AA) but less conserved modulatory domain contains putative binding sites for the numerous modulators of InsP3R activity (68). These modulators, which include Ca2+, ATP and PKA, all contribute in distinct ways to the differential Ca2+ release profiles encoded by the 3 isoforms.
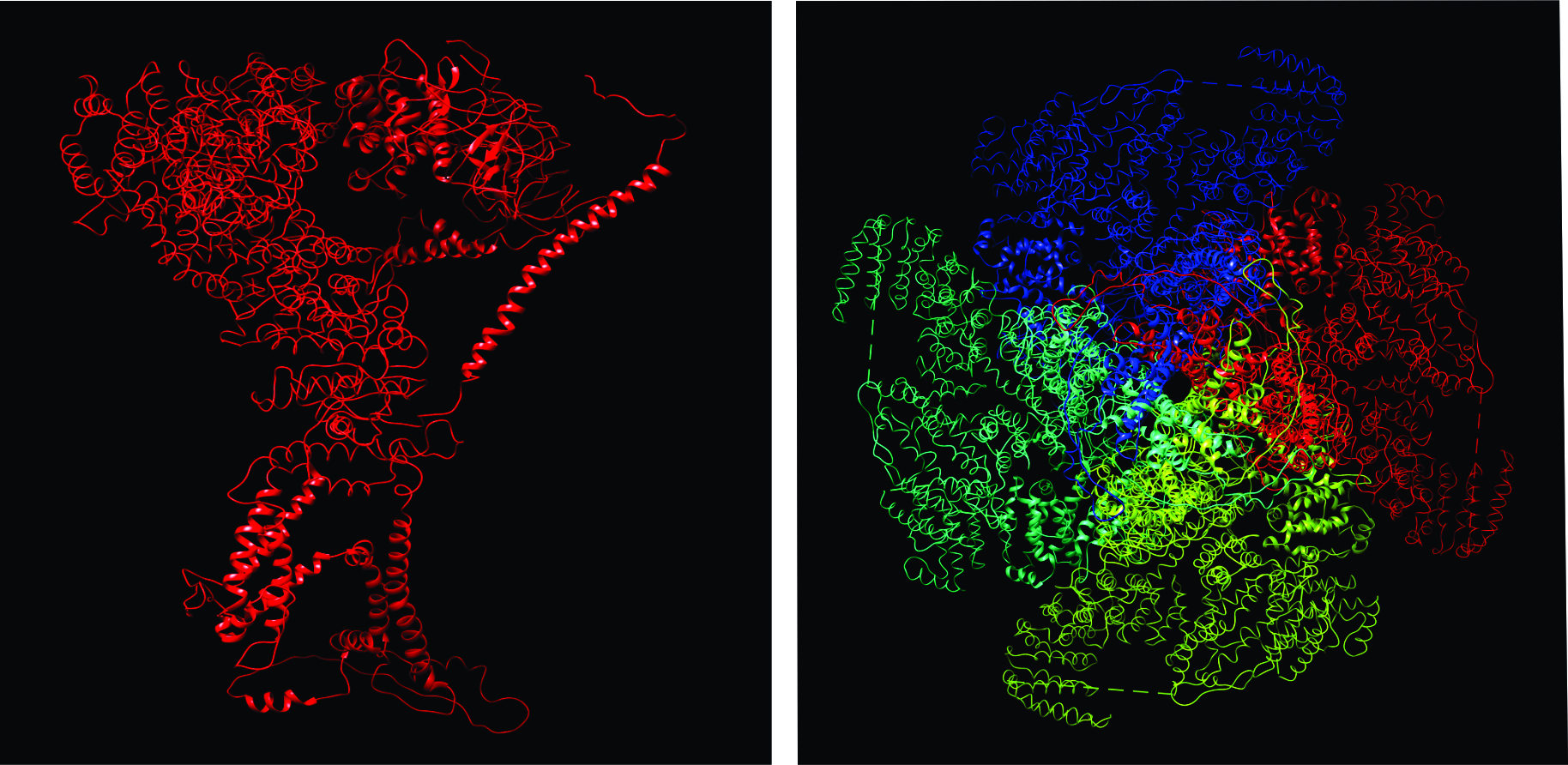
Figure 2: Illustration of R1 depicting location of suppressor domain, ligand binding core, regulation and coupling domain and channel domain (A). Structure of R1 revealed by Cryo-electron microscopy at near atomic resolution of 4.7 A (B and C). (B) is a side view along membrane plane of a R1 monomer. (C) is a tetrameric R1 viewed from cytosol. Each monomer of the tetramer are color-coded. Figures are adapted from (19)
Figure 3: (A) Permeabilized cell Ca2+ release assay in acinar cells. Isolated pancreatic acini were loaded with furaptra prior to permeabilization with β-escin. ER is loaded through SERCA upon application of Ca2+ containing buffer containing MgCl2, and ATP, as indicated by the increase in fluorescence ratio. Removal of MgCl2 deactivates SERCA and (B) addition of varying [InsP3] releases from stores as indicated by the decrease in fluorescence ratio. (C) Concentration response analysis for InsP3. EC50 for InsP3 is 0.8 µM (10)
3. Modulation of the InsP3R
Cytosolic Ca2+ is the most important regulator of InsP3Rs, modulating activity in a biphasic manner (14). Numerous putative Ca2+ binding sites have been identified, and Ca2+ has been shown to induce dramatic conformational changes in R1 (3, 4, 25, 49, 50). For the most part, studies have shown that in the presence of InsP3, low to optimal [Ca2+] (300 nM) stimulates channel activity while higher cytosolic [Ca2+] inhibits it (14). To explain this biphasic regulation, Foskett and collaborators have proposed a model in which InsP3R activity is regulated by two distinct Ca2+ binding sites: a stimulatory and an inhibitory site. Under resting conditions, the inhibitory site has a higher affinity for Ca2+ than the stimulatory site. Accordingly, this site is occupied at resting conditions and inhibits InsP3R activity. The binding of InsP3 decreases the inhibitory sites’ affinity for Ca2+, thereby allowing Ca2+ to bind to the stimulatory site and positively regulating channel activity. The downstroke of the biphasic curve is the result of Ca2+ binding to the inhibitory sites due to cytosolic [Ca2+] being elevated beyond 300 nM (57). In effect, they suggest that Ca2+ is only an essentially InsP3R co-agonist, with InsP3s sole role being simply to modulate Ca2+ sensitivities (37).
Cytosolic ATP has also been shown to differentially modulate the activity of all 3 isoforms in an allosteric manner. This mode of regulation is thought to link the metabolic status of the cell to the Ca2+ release. Specifically, studies show that R2 is only modulated by ATP at sub-maximal [InsP3] while R1 and R3 activity is affected irrespective of [InsP3], implying that ATP is required for maximal activity of R1 and R3 (8, 9). Each isoform also differs in its affinity for ATP, with R2 having 3 fold and 10 fold higher affinities than R1 and R3, respectively (8). ATP binding was originally believed to occur at Walker A like motifs (G-X-G-X-X-G) that exist in each isoform. One such motif, called ATPB, is conserved across all 3 isoforms. Additionally, R1 contains 2 other sites: ATPA and ATPC, the latter only being found in the S2- variant which is expressed in peripheral tissues (59). Recently, mutagenesis studies have demonstrated that only the ATPB site in R2 is important in mediating the modulatory effects of ATP (45) and this regulation is important for defining the sensitivity of Ca2+ release in pancreatic acinar cells (24). In contrast, the ATPB sites in R1 and R3, in addition to the ATPA and ATPC sites in R1, play no role in modulating InsP3R activity through ATP.
Further, another level of receptor regulation results from the subunit composition of InsP3R. Most cells express more than one isoform of InsP3R; as a result, different isoforms of InsP3R display overlapping expression patterns (63). Extensive studies with various experimental techniques, including cross-linking (15, 43), immunostaining (27, 33, 34, 69), and co-immunoprecipitation (1, 64), have consistently demonstrated that InsP3R can be hetero-tetrameric channels formed from at least two isoforms of InsP3R. An obvious question is what is the contribution of each subunit in a hetero-tetramer? Our lab showed that an individual subtype can either contribute equally, or dictate the Ca2+ release properties of the hetero-tetrameric InsP3R, depending on the presence and the concentrations of key regulators (ATP and Ca2+) (17). In the context of the pancreas, R2 and R3 are the predominant isoforms and form hetero-tetramers accounting for the major proportion of the InsP3R (63). A hetero-tetramer containing two R2 and two R3 is predicted to exhibit blended channel activities under optimal conditions; in contrast, R2 in the same hetero-tetramer can dictate the channel activities under suboptimal conditions (17).
Region-specific proteolysis is a novel form of regulation for all three isoforms of InsP3R. R1 has been well demonstrated to be a substrate for the intracellular cysteine proteases, caspase and calpain (28, 32). However, the functional consequences of receptor fragmentation are a subject of considerable debate. Recent evidence strongly suggests that region-specific proteolysis modifies R1 channel regulation (51, 60, 61). Specifically, stimulation of caspase or calpain fragmented R1 results in Ca2+ signals with distinct temporal characteristics and can activate alternative signaling pathways (51, 61). Further studies are necessary to guage if R2 and R3 are subject to similar regulation.
Lastly, protein kinase A (PKA) mediated phosphorylation has been shown to directly increase InsP3R Ca2+ flux and single channel activity of R1 and R2 albeit by phosphorylation at different residues. Specifically, studies show that PKA mediated phosphorylation at S1598 and S1755 on R1 and S937 on R2 significantly enhance Ca2+ release and single channel activity (7, 11, 68). Conversely, no effects on Ca2+ release through R3 have been observed after PKA stimulation, despite evidence that R3 is phosphorylated by PKA at 3 sites in vivo (52, 68). Thus far, no single channel studies have been performed on R3 to rule out any PKA mediated effects on channel activity.
InsP3Rs are also regulated and bound by numerous other kinases and accessory proteins, including cGMP protein kinase (PKG), Akt kinase, FK506 binding protein, calmodulin, CaBP, IRBIT, Bcl-2/XL, cytochrome C, RACK and Erp44 (21).
4. Tools available to study the InsP3R
A. Antibodies
A multitude of commercially available isoform specific antibodies can be utilized to probe for each isoform of the InsP3R. Additionally, there are antibodies that detect all 3 isoforms
- InsP3R1 Rabbit polyclonal (H-80) from Santa Cruz (sc-28614); epitope corresponding to AA 1894-1973 in the cytosolic modulatory domain
- InsP3R1, UC Davis/NIH NeuroMab Facility, clone L24/18; epitope against AA 2680-2749 in C-terminus
- InsP3R3 Goat polyclonal (C-20) from Santa Cruz (sc-7277); epitope corresponding to C-terminus
- InsP3R3 purified mouse monoclonal from BD Transduction Laboratories (Cat: 610313)
- InsP3R1/2/3 Rabbit polyclonal (H-300) from Santa Cruz (sc-28613); epitope corresponding to AA 2402-2701 in C-terminus of InsP3R-2 (human)
B. cDNA
Accession number:
- InsP3R1: rat, 55925609
- InsP3R2: mouse, 60593031
- InsP3R3: rat, 6981109
C. Cell Lines
- SHSYFY Neuroblastoma: 99% InsP3R1 (63)
- AR42J Rat pancreatoma: 86% InsP3R2; 12% InsP3R1; 2% InsP3R3 (63)
- RINM5F Mouse insulinoma: 96% InsP3R3; 4% InsP3R1(63)
DT40 triple knockout cell lines: Kurosaki and colleagues have generated a DT40 chicken B lymphocyte cell line with the endogenous InsP3Rs stably knocked out (55). These triple knockout cells can subsequently be stably transfected with constructs encoding individual mammalian InsP3R isoforms, allowing the study of activity and regulation of each isoform in isolation. These cell lines are available on request from our laboratory.
HEK triple knockout cell line: David Yule and colleagues have generated HEK triple knockout cell line with the endogenous InsP3Rs stably knockout (2). In addition to DT40 triple knockout cell line, HEK triple knockout cell line provides an alternative mammalian InsP3R null background system for research.
D. Mouse Lines
InsP3R-1 knockout mice (39)
InsP3R-2 (35) and R-3 single and double knockout mice (22)
E. Agonists/Antagonists/
Agonists
Commercially available InsP3: D-myo-inositol 1,4,5-trisphoshate hexapotassium salt; Enzo Life Sciences (cat: ALX-307-00)
Cells are often stimulated with Gq coupled GPCR agonists to stimulate InsP3 production. Agonists include acetylcholine, trypsin and cholecystokinin.
Antagonists
Heparin (66); Caffeine (13); 2-Aminoethoxydiphenyl borate (2-APB) (38, 41); Xestospongin (23)
F. Techniques used to study InsP3R function
See review by Betzenhauser MJ., Wagner LE., Won JW and Yule DI. (2008) Studying isoform-specific inositol 1,4,5-trisphosphate receptor function and regulation. Methods 46, pp: 177-182. (10)
Betzenhauser, Matthew J., Won, Jong Hak, Park, Hyungseo and Yule, David I. (2011). Measurement of Intracellular Calcium Concentration in Pancreatic Acini. The Pancreapedia: Exocrine Pancreas Knowledge Base, DOI:10.3998/panc.2011.34
5. References
- Alzayady KJ, Wagner LE, 2nd, Chandrasekhar R, Monteagudo A, Godiska R, Tall GG, et al. Functional inositol 1,4,5-trisphosphate receptors assembled from concatenated homo- and heteromeric subunits. J Biol Chem 288(41): 29772-29784,2013. PMID: 23955339.
- Alzayady KJ, Wang L, Chandrasekhar R, Wagner LE, 2nd, Van Petegem F and Yule DI. Defining the stoichiometry of inositol 1,4,5-trisphosphate binding required to initiate Ca2+ release. Sci Signal 9(422): ra35,2016. PMID: 27048566.
- Anyatonwu G and Joseph SK. Surface accessibility and conformational changes in the N-terminal domain of type I inositol trisphosphate receptors: studies using cysteine substitution mutagenesis. J Biol Chem 284(12): 8093-8102,2009. PMID: 19141613.
- Anyatonwu G, Khan MT, Schug ZT, da Fonseca PC, Morris EP and Joseph SK. Calcium-dependent conformational changes in inositol trisphosphate receptors. J Biol Chem 285(32): 25085-25093,2010. PMID: 20530483.
- Ashby MC, Camello-Almaraz C, Gerasimenko OV, Petersen OH and Tepikin AV. Long distance communication between muscarinic receptors and Ca2+ release channels revealed by carbachol uncaging in cell-attached patch pipette. J Biol Chem 278(23): 20860-20864,2003. PMID: 12657637
- Bayerdorffer E, Streb H, Eckhardt L, Haase W and Schulz I. Characterization of calcium uptake into rough endoplasmic reticulum of rat pancreas. J Membr Biol 81(1): 69-82,1984. PMID: 6208363.
- Betzenhauser MJ, Fike JL, Wagner LE, 2nd and Yule DI. Protein kinase A increases type-2 inositol 1,4,5-trisphosphate receptor activity by phosphorylation of serine 937. J Biol Chem 284(37): 25116-25125,2009. PMID: 19608738.
- Betzenhauser MJ, Wagner LE, 2nd, Iwai M, Michikawa T, Mikoshiba K and Yule DI. ATP modulation of Ca2+ release by type-2 and type-3 inositol (1, 4, 5)-triphosphate receptors. Differing ATP sensitivities and molecular determinants of action. J Biol Chem 283(31): 21579-21587,2008. PMID: 18505727.
- Betzenhauser MJ, Wagner LE, 2nd, Park HS and Yule DI. ATP regulation of type-1 inositol 1,4,5-trisphosphate receptor activity does not require walker A-type ATP-binding motifs. J Biol Chem 284(24): 16156-16163,2009. PMID: 19386591.
- Betzenhauser MJ, Wagner LE, 2nd, Won JH and Yule DI. Studying isoform-specific inositol 1,4,5-trisphosphate receptor function and regulation. Methods 46(3): 177-182,2008. PMID: 18929664.
- Betzenhauser MJ and Yule DI. Regulation of inositol 1,4,5-trisphosphate receptors by phosphorylation and adenine nucleotides. Curr Top Membr 66C: 273-298,2010. PMID: 22353484.
- Bezprozvanny I. The inositol 1,4,5-trisphosphate receptors. Cell Calcium 38(3-4): 261-272,2005. PMID: 16102823.
- Bezprozvanny I, Bezprozvannaya S and Ehrlich BE. Caffeine-induced inhibition of inositol(1,4,5)-trisphosphate-gated calcium channels from cerebellum. Mol Biol Cell 5(1): 97-103,1994. PMID: 8186468.
- Bezprozvanny I, Watras J and Ehrlich BE. Bell-shaped calcium-response curves of Ins(1,4,5)P3- and calcium-gated channels from endoplasmic reticulum of cerebellum. Nature 351(6329): 751-754,1991. PMID: 1648178.
- Boehning D and Joseph SK. Direct association of ligand-binding and pore domains in homo- and heterotetrameric inositol 1,4,5-trisphosphate receptors. EMBO J 19(20): 5450-5459,2000. PMID: 11032812.
- Boehning D, Mak DO, Foskett JK and Joseph SK. Molecular determinants of ion permeation and selectivity in inositol 1,4,5-trisphosphate receptor Ca2+ channels. J Biol Chem 276(17): 13509-13512,2001. PMID: 11278266.
- Chandrasekhar R, Alzayady KJ, Wagner LE, 2nd and Yule DI. Unique Regulatory Properties of Heterotetrameric Inositol 1,4,5-Trisphosphate Receptors Revealed by Studying Concatenated Receptor Constructs. J Biol Chem 291(10): 4846-4860,2016. PMID: 26755721.
- Chandrasekhar R, Alzayady KJ and Yule DI. Using concatenated subunits to investigate the functional consequences of heterotetrameric inositol 1,4,5-trisphosphate receptors. Biochem Soc Trans 43(3): 364-370,2015. PMID: 26009177.
- Fan G, Baker ML, Wang Z, Baker MR, Sinyagovskiy PA, Chiu W, et al. Gating machinery of InsP3R channels revealed by electron cryomicroscopy. Nature 527(7578): 336-341,2015. PMID: 26458101.
- Fogarty KE, Kidd JF, Tuft DA and Thorn P. Mechanisms underlying InsP(3)-evoked global Ca2+ signals in mouse pancreatic acinar cells. J Physiol 526(3): 515-526,2000. DOI 10.1111/j.1469-7793.2000.t01-1-00515.x
- Foskett JK, White C, Cheung KH and Mak DO. Inositol trisphosphate receptor Ca2+ release channels. Physiol Rev 87(2): 593-658,2007. PMID: 17429043.
- Futatsugi A, Nakamura T, Yamada MK, Ebisui E, Nakamura K, Uchida K, et al. IP3 receptor types 2 and 3 mediate exocrine secretion underlying energy metabolism. Science 309(5744): 2232-2234,2005. PMID: 16195467.
- Gafni J, Munsch JA, Lam TH, Catlin MC, Costa LG, Molinski TF, et al. Xestospongins: potent membrane permeable blockers of the inositol 1,4,5-trisphosphate receptor. Neuron 19(3): 723-733,1997. PMID: 9331361.
- Giovannucci DR, Bruce JI, Straub SV, Arreola J, Sneyd J, Shuttleworth TJ, et al. Cytosolic Ca2+ and Ca2+-activated Cl- current dynamics: insights from two functionally distinct mouse exocrine cells. J Physiol 540(Pt 2): 469-484,2002. PMID: 11956337.
- Hamada K, Miyata T, Mayanagi K, Hirota J and Mikoshiba K. Two-state conformational changes in inositol 1,4,5-trisphosphate receptor regulated by calcium. J Biol Chem 277(24): 21115-21118,2002. PMID: 11980893.
- Hamada K, Miyatake H, Terauchi A and Mikoshiba K. IP3-mediated gating mechanism of the IP3 receptor revealed by mutagenesis and X-ray crystallography. Proc Natl Acad Sci U S A 114(18): 4661-4666,2017. PMID: 28416699.
- Hirata K, Pusl T, O'Neill AF, Dranoff JA and Nathanson MH. The type II inositol 1,4,5-trisphosphate receptor can trigger Ca2+ waves in rat hepatocytes. Gastroenterology 122(4): 1088-1100,2002. PMID: 11910359.
- Hirota J, Furuichi T and Mikoshiba K. Inositol 1,4,5-trisphosphate receptor type 1 is a substrate for caspase-3 and is cleaved during apoptosis in a caspase-3-dependent manner. J Biol Chem 274(48): 34433-34437,1999. PMID: 10567423.
- Iwai M, Michikawa T, Bosanac I, Ikura M and Mikoshiba K. Molecular basis of the isoform-specific ligand-binding affinity of inositol 1,4,5-trisphosphate receptors. J Biol Chem 282(17): 12755-12764,2007. PMID: 17327232.
- Joseph SK, Boehning D, Pierson S and Nicchitta CV. Membrane insertion, glycosylation, and oligomerization of inositol trisphosphate receptors in a cell-free translation system. J Biol Chem 272(3): 1579-1588,1997. PMID: 8999831.
- Kasai H and Augustine GJ. Cytosolic Ca-2+ Gradients Triggering Unidirectional Fluid Secretion from Exocrine Pancreas. Nature 348(6303): 735-738,1990. PMID: 1701852
- Kopil CM, Vais H, Cheung KH, Siebert AP, Mak DO, Foskett JK, et al. Calpain-cleaved type 1 inositol 1,4,5-trisphosphate receptor (InsP(3)R1) has InsP(3)-independent gating and disrupts intracellular Ca(2+) homeostasis. J Biol Chem 286(41): 35998-36010,2011. PMID: 21859719.
- Kruglov EA, Gautam S, Guerra MT and Nathanson MH. Type 2 inositol 1,4,5-trisphosphate receptor modulates bile salt export pump activity in rat hepatocytes. Hepatology 54(5): 1790-1799,2011. PMID: 21748767.
- Lee MG, Xu X, Zeng W, Diaz J, Kuo TH, Wuytack F, et al. Polarized expression of Ca2+ pumps in pancreatic and salivary gland cells. Role in initiation and propagation of [Ca2+]i waves. J Biol Chem 272(25): 15771-15776,1997. PMID: 9188473.
- Li X, Zima AV, Sheikh F, Blatter LA and Chen J. Endothelin-1-induced arrhythmogenic Ca2+ signaling is abolished in atrial myocytes of inositol-1,4,5-trisphosphate(IP3)-receptor type 2-deficient mice. Circ Res 96(12): 1274-1281,2005. PMID: 15933266.
- Lur G, Sherwood MW, Ebisui E, Haynes L, Feske S, Sutton R, et al. InsP(3)receptors and Orai channels in pancreatic acinar cells: co-localization and its consequences. Biochem J 436(2): 231-239,2011. PMID: 21568942.
- Mak DO, McBride S and Foskett JK. Inositol 1,4,5-trisphosphate [correction of tris-phosphate] activation of inositol trisphosphate [correction of tris-phosphate] receptor Ca2+ channel by ligand tuning of Ca2+ inhibition. Proc Natl Acad Sci U S A 95(26): 15821-15825,1998. PMID: 9861054.
- Maruyama T, Kanaji T, Nakade S, Kanno T and Mikoshiba K. 2APB, 2-aminoethoxydiphenyl borate, a membrane-penetrable modulator of Ins(1,4,5)P3-induced Ca2+ release. J Biochem 122(3): 498-505,1997. PMID: 9348075.
- Matsumoto M, Nakagawa T, Inoue T, Nagata E, Tanaka K, Takano H, et al. Ataxia and epileptic seizures in mice lacking type 1 inositol 1,4,5-trisphosphate receptor. Nature 379(6561): 168-171,1996. PMID: 8538767.
- Mignery GA, Sudhof TC, Takei K and Decamilli P. Putative receptor for inositol 1,4,5-trisphosphate similar to ryanodine receptor. Nature 342(6246): 192-195,1989. PMID: 2554146
- Missiaen L, Callewaert G, De Smedt H and Parys JB. 2-Aminoethoxydiphenyl borate affects the inositol 1,4,5-trisphosphate receptor, the intracellular Ca2+ pump and the non-specific Ca2+ leak from the non-mitochondrial Ca2+ stores in permeabilized A7r5 cells. Cell Calcium 29(2): 111-116,2001. PMID: 11162848.
- Nathanson MH, Padfield PJ, O'Sullivan AJ, Burgstahler AD and Jamieson JD. Mechanism of Ca2+ wave propagation in pancreatic acinar cells. J Biol Chem 267(25): 18118-18121,1992. PMID: 1517244.
- Onoue H, Tanaka H, Tanaka K, Doira N and Ito Y. Heterooligomer of type 1 and type 2 inositol 1, 4, 5-trisphosphate receptor expressed in rat liver membrane fraction exists as tetrameric complex. Biochem Biophys Res Commun 267(3): 928-933,2000. PMID: 10673393.
- Pantazaka E and Taylor CW. Targeting of inositol 1,4,5-trisphosphate receptor to the endoplasmic reticulum by its first transmembrane domain. Biochemical Journal 425: 61-69,2010. PMID: 19845505
- Park HS, Betzenhauser MJ, Won JH, Chen J and Yule DI. The type 2 inositol (1,4,5)-trisphosphate (InsP3) receptor determines the sensitivity of InsP3-induced Ca2+ release to ATP in pancreatic acinar cells. J Biol Chem 283(38): 26081-26088,2008. PMID: 18658132.
- Parker AKT, Gergely FV and Taylor CW. Targeting of inositol 1,4,5-trisphosphate receptors to the endoplasmic reticulum by multiple signals within their transmembrane domains. J Biol Chem 279(22): 23797-23805,2004. PMID: 15033979
- Patel S, Joseph SK and Thomas AP. Molecular properties of inositol 1,4,5-trisphosphate receptors. Cell Calcium 25(3): 247-264,1999. PMID: 10378086.
- Ramos-Franco J, Galvan D, Mignery GA and Fill M. Location of the permeation pathway in the recombinant type 1 inositol 1,4,5-trisphosphate receptor. J Gen Physiol 114(2): 243-250,1999. PMID: 10436000.
- Sienaert I, De Smedt H, Parys JB, Missiaen L, Vanlingen S, Sipma H, et al. Characterization of a cytosolic and a luminal Ca2+ binding site in the type I inositol 1,4,5-trisphosphate receptor. J Biol Chem 271(43): 27005-27012,1996. PMID: 8900188.
- Sienaert I, Missiaen L, De Smedt H, Parys JB, Sipma H and Casteels R. Molecular and functional evidence for multiple Ca2+-binding domains in the type 1 inositol 1,4,5-trisphosphate receptor. J Biol Chem 272(41): 25899-25906,1997. PMID: 9325322.
- Sneyd J, Han JM, Wang L, Chen J, Yang X, Tanimura A, et al. On the dynamical structure of calcium oscillations. Proc Natl Acad Sci U S A 114(7): 1456-1461,2017. PMID: 28154146.
- Soulsby MD and Wojcikiewicz RJ. The type III inositol 1,4,5-trisphosphate receptor is phosphorylated by cAMP-dependent protein kinase at three sites. Biochem J 392(Pt 3): 493-497,2005. PMID: 16107208.
- Streb H, Bayerdorffer E, Haase W, Irvine RF and Schulz I. Effect of inositol-1,4,5-trisphosphate on isolated subcellular fractions of rat pancreas. J Membr Biol 81(3): 241-253,1984. PMID: 6334162.
- Streb H, Irvine RF, Berridge MJ and Schulz I. Release of Ca2+ from a nonmitochondrial intracellular store in pancreatic acinar cells by inositol-1,4,5-trisphosphate. Nature 306(5938): 67-69,1983. PMID: 6605482.
- Sugawara H, Kurosaki M, Takata M and Kurosaki T. Genetic evidence for involvement of type 1, type 2 and type 3 inositol 1,4,5-trisphosphate receptors in signal transduction through the B-cell antigen receptor. EMBO J 16(11): 3078-3088,1997. PMID: 9214625.
- Supattapone S, Worley PF, Baraban JM and Snyder SH. Solubilization, purification, and characterization of an inositol trisphosphate receptor. J Biol Chem 263(3): 1530-1534,1988. PMID: 2826483.
- Taylor CW and Laude AJ. IP3 receptors and their regulation by calmodulin and cytosolic Ca2+. Cell Calcium 32(5-6): 321-334,2002. PMID: 12543092.
- Thorn P, Lawrie AM, Smith PM, Gallacher DV and Petersen OH. Local and global cytosolic Ca2+ oscillations in exocrine cells evoked by agonists and inositol trisphosphate. Cell 74(4): 661-668,1993. PMID: 8395347.
- Wagner LE, 2nd, Betzenhauser MJ and Yule DI. ATP binding to a unique site in the type-1 S2- inositol 1,4,5-trisphosphate receptor defines susceptibility to phosphorylation by protein kinase A. J Biol Chem 281(25): 17410-17419,2006. PMID: 16621795.
- Wang L, Alzayady KJ and Yule DI. Proteolytic fragmentation of inositol 1,4,5-trisphosphate receptors: a novel mechanism regulating channel activity? J Physiol 594(11): 2867-2876,2016. PMID: 26486785.
- Wang L, Wagner LE, 2nd, Alzayady KJ and Yule DI. Region-specific proteolysis differentially regulates type 1 inositol 1,4,5-trisphosphate receptor activity. J Biol Chem 292(28): 11714-11726,2017. PMID: 28526746.
- Williams JA and Yule DI. Stimulus-secretion coupling in pancreatic acinar cells. . Physiology of the Gastrointestinal Tract. LR Johnson. 2: 2197, 2012.
- Wojcikiewicz RJ. Type I, II, and III inositol 1,4,5-trisphosphate receptors are unequally susceptible to down-regulation and are expressed in markedly different proportions in different cell types. J Biol Chem 270(19): 11678-11683,1995. PMID: 7744807.
- Wojcikiewicz RJ and He Y. Type I, II and III inositol 1,4,5-trisphosphate receptor co-immunoprecipitation as evidence for the existence of heterotetrameric receptor complexes. Biochem Biophys Res Commun 213(1): 334-341,1995. PMID: 7639754.
- Won JH, Cottrell WJ, Foster TH and Yule DI. Ca2+ release dynamics in parotid and pancreatic exocrine acinar cells evoked by spatially limited flash photolysis. Am J Physiol Gastrointest Liver Physiol 293(6): G1166-1177,2007. PMID: 17901163.
- Yamamoto H, Kanaide H and Nakamura M. Heparin specifically inhibits the inositol 1,4,5-trisphosphate-induced Ca2+ release from skinned rat aortic smooth muscle cells in primary culture. Naunyn Schmiedebergs Arch Pharmacol 341(4): 273-278,1990. PMID: 2333098.
- Yoshikawa F, Morita M, Monkawa T, Michikawa T, Furuichi T and Mikoshiba K. Mutational analysis of the ligand binding site of the inositol 1,4,5-trisphosphate receptor. J Biol Chem 271(30): 18277-18284,1996. PMID: 8663526.
- Yule DI, Betzenhauser MJ and Joseph SK. Linking structure to function: Recent lessons from inositol 1,4,5-trisphosphate receptor mutagenesis. Cell Calcium 47(6): 469-479,2010. PMID: 20510450.
- Yule DI, Ernst SA, Ohnishi H and Wojcikiewicz RJ. Evidence that zymogen granules are not a physiologically relevant calcium pool. Defining the distribution of inositol 1,4,5-trisphosphate receptors in pancreatic acinar cells. J Biol Chem 272(14): 9093-9098,1997. PMID: 9083036.