Entry Version:
Citation:
Pancreapedia: Exocrine Pancreas Knowledge Base, DOI: 10.3998/panc.2011.18
Attachment | Size |
---|---|
![]() | 357.22 KB |
1. General Information
Background
Hydrogen sulfide (H2S; CAS No. 7783-06-4) is a colorless, flammable gas with a characteristic odor of rotten eggs. It is produced naturally and as a result of human activity. Natural sources account for about 90% of the total H2S in the atmosphere (56). Concentrations of H2S in ambient air as a result of natural sources have been estimated to be between 0.14 and 0.4 µg/m3 (56). The odor threshold of H2S is 0.011 mg/m3. Human exposure at ranges between 5 and 700 mg/m3 have reported to result in ocular, respiratory and neurological complications. At concentrations > 700mg/m3, incidences of death have been reported (5).
Endogenous H2S synthesis and metabolism
Apart from environmental and bacterial sources, mammals too are capable of synthesizing H2S. Endogenous levels of H2S have been measured in the circulatory system with rat serum being reported to contain ~46uM H2S (67). H2S synthesizing activity has also been shown in rat tissue extracts of liver, kidney, heart, brain, small intestine, skeletal muscle and pancreas (22). H2S can be hydrolyzed to hydrosulfide and sulfide ions. In an aqueous solution, about one third of H2S remains undissociated at pH 7.4. H2S is permeable to plasma membranes as its solubility in lipophilic solvents is ~ fivefold greater than in water (53).
Endogenous H2S can be synthesized via the desulfuration of cystine/cysteine by three enzymes; Cystathionine beta synthase (CBS; EC 4.2.1.22), Cystathionine gamma Lyase (CSE; EC 4.4.1.1) and mercaptopyruvate sulfurtransferase (MST; EC 2.8.1.2) MST is found both in the mitochondria and cytosol while CBS and CSE are mainly produced in the cytosol. (22). Among the 3 enzymes, MST contributes the least towards endogenous H2S production (36, 42) while CBS seems to be the main H2S-forming enzyme in the CNS and CSE is the main H2S-forming enzyme in the cardiovascular system (34). In mice, CSE expression has been detected mainly in the liver and kidney, and in lower abundance in adipose tissue, stomach, small intestine, brain, heart and lung (20). CBS expression has been found in all parts of the brain, liver and pancreas (4, 38). In mouse pancreas, CBS is ubiquitously distributed but CSE was found mostly in the exocrine and in very small amounts in the freshly prepared islets. However, high glucose increased the CSE expression in the beta-cells (23).
Both CSE and CBS are pyridoxal 5’-phosphate dependant enzymes. CBS is able to synthesize H2S directly by substituting the thiol group L-cysteine with a variety of thiol compounds to form H2S and the corresponding thioether. CSE on the other hand catalyzes the desulfhydration of cystine which results in pyruvate, NH4+, and thiocysteine. Thiocysteine then reacts with cysteine or other thiols to form H2S (42) (Figure 1) The metabolism of H2S can be divided to 3 distinct pathways: oxidation to sulfate, methylation, and reaction with metallo or disulfide containing proteins (5). Oxidation of sulfide to sulfate and subsequent excretion by the kidney is thought to represent the major metabolic and secretory pathway.
Physiological and pathological functions
Rapid development in the H2S field has revealed numerous possible physiological and pathological roles for H2S. Below is a brief introduction into the current areas in H2S have been discovered to play a role.
Neuromodulation
Physiological concentrations of H2S selectively enhance NMDA receptor-mediated responses and facilitate the induction of hippocampal long term potentiation (1) as well as regulate the release of the corticotrophin-releasing hormone from the hypothalamus (14). An antinociceptive role of H2S has been reported in colorectal distensions in rats mediated by KATP channels and NO (15). Subsequent studies have however, reported a pronociceptive role for H2S through the sensitization/ activation of T-type Ca2+ channels (31, 16).
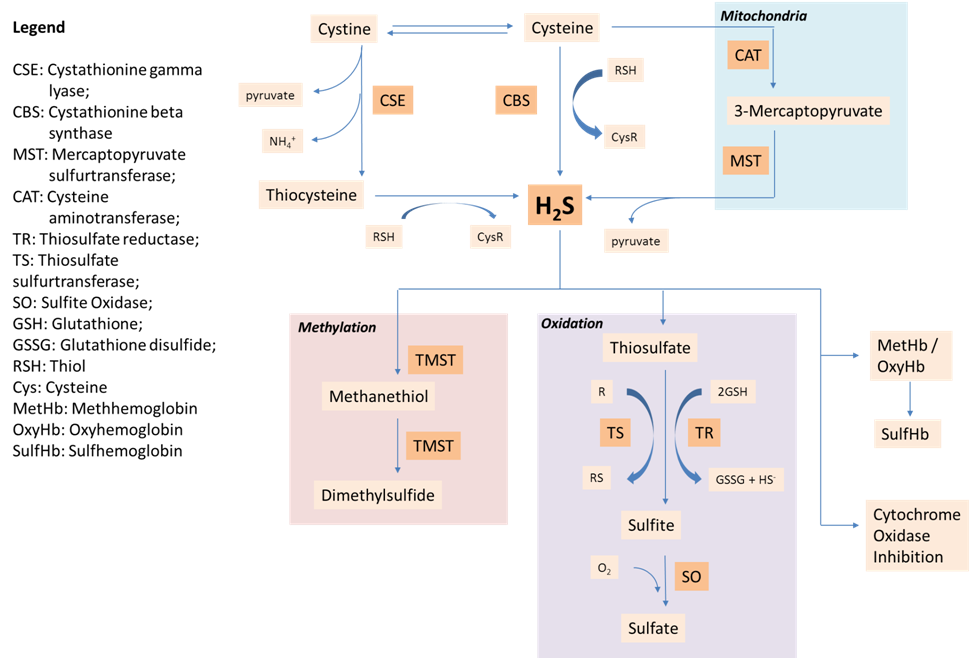
Figure 1 . Endogenous H2S synthesis and metabolism
Cardiovascular function
H2S has been demonstrated to be a vasoactive factor that relaxes rat thoracic aorta and portal vein, and guinea pig ileum in a concentration dependent manner (18). This action has been shown to be mediated by activation of KATP channels resulting in a hyperpolarized membrane leading to smooth muscle relaxation (67). A growing body of evidence suggests that H2S preconditioning protects against myocardial injury (9, 21, 40, 41)
Interaction with reactive- oxygen and nitrogen species
H2S has been shown to possess scavenging properties towards peroxynitrite (54) and hypochlorous acid (27, 55). An indirect anti-oxidant effect was observed in primary neuron cultures where H2S was found to increase glutathione levels by enhancing the activity of gamma-glutamylcysteine synthetase and up-regulating cystine transport (26). H2S has also been shown to interact with reactive oxygen species from activated neutrophils resulting in its oxidation to sulfite which is toxic at high levels (32).
Inflammation
The role of H2S in inflammation is still a matter of debate. In most of the studies, H2S has been shown to be pro-inflammatory. This was observed in various animal models of inflammation such as LPS induced endotoxemia (28), pancreatitis (8), cecal-ligation and puncture induced sepsis (65), hemorrhagic shock (33), burns injury (66) and cisplatin induced renal injury (13). In these studies, pre-treatment with inhibitor of endogenous H2S synthesis, PAG afforded protection while administration of H2S in the form of NaHS resulted in exacerbation.
On the other hand, studies have reported an anti-inflammatory effect on gastritis and colitis using Lawesson reagent as a sulfide donor as well as NaHS at a low dose (51, 52). A slow releasing H2S molecule, GYY4137 has also been shown to protect mice against LPS induced endotoxemia (30) by the same author that previously showed pro-inflammatory effect using NaHS (28). H2S releasing drugs have also been shown to have anti-inflammatory effect attributed to the sulfide moiety and not the parent drug, diclofenac (6, 29). Conflicting observations have also been reported on the effect of H2S on leukocyte rolling and adhesion. Studies have shown that H2S both positively and negatively regulate leukocyte activation and migration. (3, 12, 64, 65, 68). It is becoming apparent that H2S has a multifaceted role in inflammation and current studies vary in opinions largely based on the dose, rate of release, and type of H2S donor used.
2. Pancreatic Information
1. H2S and Insulin secretion
Mobilization and secretion of insulin-containing vesicles into the extracellular space is regulated by cellular Ca movements via the regulation of the voltage gated Ca channel which is activated based on membrane polarization. H2S which is actively synthesized in the pancreas has been shown to activate KATP channels in smooth muscle cells which regulate membrane polarization. Therefore it has become of interest to study the possible role of H2S in insulin mobilization.
Freshly prepared pancreatic islet and related cell lines are reported to express H2S synthesizing enzymes, CSE and CBS (24, 60, 63). H2S synthesizing rates were reported in INS-1E cells at 12 nmole/g/min in 5mM glucose (60) and fresh rat pancreas extract at 38 nmole/g/30min (63) and 8 nmole/g/min (57). Increased glucose concentration (20mM) in INS-1E cells resulted in a decrease in H2S synthesis to 6 nmole/g/min (60). Altered H2S synthesis was also observed in fresh pancreatic extracts of Streptozotocin-diabetic and Zucker diabetic fatty rats which increased to 65 nmole/g/30min (63) and 12 nmole/g/min (57) respectively. However the identified enzyme that was responsible for this observed increase in H2S synthesis differed between these two models of diabetes. In the Streptozocin-diabetic rats, the increased H2S synthesis was attributed due to an increased CBS expression of up to 2-fold (63), while in Zucker diabetic fatty rats it was due to increased CSE expression (57).
These changes in H2S levels affect the amount of insulin secreted, the general consensus being H2S functions as an inhibitor to insulin secretion. It has been reported that exposure of beta islet cells to exogenous or increased endogenous H2S significantly reduced glucose-induced insulin secretion (2, 24, 57, 63) The observed suppression of insulin secretion by H2S has been associated with the opening of KATP channels. The reported effective dose of NaHS (exogenous source of H2S) that resulted in activation of KATP channels and reduced insulin secretion in INS-1E and HIT-T15 cells was 100uM (60, 2). This was abolished following pre-treatment with a KATP channel blocker, glibenclamide (2). Similar findings were reported in freshly prepared islet cells from rats (57). Further molecular mechanism studies revealed that exogenous H2S prevents Ca influx (2) and oscillation (24) which are essential to insulin secretion (37)
2. H2S and pancreatic cell death
H2S has been reported to induce apoptosis in both exocrine and endocrine cells of the pancreas. Pancreatic acinar cells treated with 10 uM NaHS for 3 hours showed positive Annexin V staining (10). This was accompanied with the activation of effector caspase -3 and initiator caspase- 8 and 9 as well as loss of mitochondrial membrane integrity and release of cytochrome C. Additionally pro-apoptotic proteins Bax was upregulated while anti-apoptotic protein FLIP was downregulated. INS-1E cells stimulated with 100 uM H2S (pure H2S gas) and CSE over-expressing INS-1E cells were found to be apoptotic at 12 hours and 48 hours respectively (59). The authors reported an inhibition of ERK 1/2 and activation of p38 MAPK as well as upregulation of ER stress regulators; BiP and CHOP following H2S stimulation. Inhibition of p38 MAPK inhibited expression of BiP and CHOP leading to a decrease in H2S mediated apoptosis in INS-1E cells.
Interestingly, H2S has also been shown to have anti-apoptotic effect on pancreatic endocrine cells. 100 uM NaHS and 3 mM L-Cysteine suppressed freshly prepared mouse islet cell apoptosis induced with high glucose (20 mM) for 18 hours (23). H2S has also been shown to protect freshly prepared mouse islet cells against apoptosis induced by palmitate, H2O2 and cytokine mixture (TNF-a, IL-1b and INF-g) but did not protect against thapsigarin and tunicamycin (49). Similar findings were found with MIN6 cells (23). Administration of NaHS was found to reduce oxidative stress by increasing glutathione content (23) and suppression of ROS production (49) in MIN6 cells following insult.
3. H2S modulates pancreatic nociception
NaHS was shown to be pronociceptive when administered into the pancreatic duct of rats at 500 nmole /rat (35, 16). Nociception was determined by expression of Fos proteins as well as phosphorylation of ERK in the spinal dorsal horns of the animals. The upregulation of Fos and phosphorylation of ERK was abolished following pre-treatment with Mibefadril, a T-type Ca2+ channel blocker. In the caerulein induced pancreatitis model, the prevention of pancreatitis associated allodynia/hyperalgesia has been reported by pre-treatment with a CSE blocker (PAG) and treatment with a T-type Ca2+ channel blocker (Mibefadril) (35).
4. H2S regulates the severity of inflammatory response in acute pancreatitis and associated lung injury
Caerulein induced acute pancreatitis upregulates CSE expression and H2S production whereas inhibition of endogenous H2S formation with DL-propargylglycine (PAG), a CSE inhibitor, reduces the severity of caerulein-induced acute pancreatitis and affords protection against the associated lung injury in mice (8). H2S donor, NaHS provoked inflammation in both mouse pancreatic acinar cells (46) and primary human monocyte cells (68). Therefore, observations using H2S donor in murine cells could still be extrapolated to human cells, and may provide useful guidance on the potential therapeutic benefits of using H2S in inflammatory conditions.
Studies have suggested that H2S has both pro and anti-inflammatory properties, depending largely on the experimental conditions and the cell type under scrutiny. The mechanism through which H2S is able to promote and delay inflammatory response still remains to be fully elucidated. However, it has been proposed that low concentrations (NaHS- 5 and 10 µM) (48) of H2S, have a protective effect. On the other hand higher concentrations (100 µM) (46) induce inflammation in the acini. Another H2S donor ACS15, is a H2S releasing derivative of Diclofenac. ACS15 reduced lung inflammation without having any effect on pancreatic injury (6). In addition Nishimura et al showed that pancreatic NaHS/ H2S most probably targets T-type Ca(2+) channels, leading to nociception, and that endogenous H2S produced by CSE and possibly T-type Ca(2+) channels are involved in pancreatitis-related pain (35).
Altering endogenous or exogenous H2S may have unanticipated effects because H2S has a wide array of actions for example H2S regulates chemokines, cytokines and adhesion molecule expression and exerts explicit biphasic effect in acute pancreatitis and associated lung injury (Fig 2) (39, 45). At present, there are several pathways that appear to be involved in the potential effects of H2S on acute pancreatitis. Although the mechanism of the pro-inflammatory effect of H2S has not yet been fully investigated, it is likely that H2S stimulates neutrophil adhesion to caerulein treated pancreatic acinar cells and subsequent intracellular adhesion molecule (ICAM)-1 upregulation through Src family kinases (44).
One of the major messengers in the upregulation of ICAM-1, and neutrophil adhesion, is nuclear transcription factor-kB (NF-kB). NF-kB is a transcription factor that translocates to the cell nucleus on activation by H2S, whereupon it acts as a signal for increased transcription of ICAM-1. This increase in ICAM-1 mRNA was consistent with Western blot analysis of increased NF-kB in caerulein treated pancreatic acinar cells. The mechanism of this enhanced ICAM-1 mRNA appears to relate to IkB-α, since H2S donor inhibits IkB-α phosphorylation. IkB-α is the inhibitory subunit of NF-kB and thus inhibition of IkB-α activates the expression of NF-kB, effectively upregulating ICAM-1 expression on pancreatic acinar cells. In caerulein treated pancreatic acini, PAG reduces ICAM-1 expression which led to decrease in neutrophil adhesion to acini via Src family kinases and NF-kB (44).
Another potential mechanism of action of H2S is its interaction with the neuropeptide Substance P in both an in vivo (7) and an in vitro model of acute pancreatitis (46). As a consequence of this interaction Substance P is upregulated via activation of TLR4 and NF-kB pathway (7). Blockade of H2S biosynthesis by PAG and PPTA deficiency attenuates H2S induced TLR4 and NF-kB pathway possibly by stimulation of IkB-α phosphorylation (Fig 2) (47).
Recently, we analyzed the mechanism by which H2S reduces caerulein induced inflammation in pancreatic acini. We found that H2S activation of the PI3K/AKT pathway negatively regulates the intracellular signaling pathway, ERK, transcription factor NF-kB and the cytokines in caerulein treated pancreatic acinar cells (Fig 2) (48). Activation of PI3K/AKT signaling mediates the anti-inflammatory effects in inflammation (62).
Differences may exist in the mechanisms by which H2S induces inflammation. It is also important to realize that the concentration of H2S donor used may not necessarily reflect the concentration of H2S to which the cells are exposed. Equivalent concentrations of different H2S donors may liberate H2S to different extents or at different rates. Therefore, the concentration of free H2S in the vicinity of the cells at any given time may vary, and the H2S concentration in the system needs to be measured in order to directly compare different H2S donors. The vast majority of work on this subject has been carried out using in vitro and in vivo systems, utilizing animals. How the results obtained in these systems relate to the in vivo situation during inflammation in humans still largely remains to be determined, but recent studies in mice show that H2S is a promising candidate for treatment or prevention of inflammatory conditions. Further studies are required to elucidate completely the mechanism of action of H2S on inflammation, in order to identify potential targets for the treatment of human inflammatory conditions and to evaluate the sources of H2S that provide greatest therapeutic potential.
Summary
H2S is produced by three enzymes, CSE, CBS and MST which are present in most mammals including humans. Both CBS and CSE are expressed in the pancreas. H2S has been found to have both physiological and pathological roles in the pancreas. Currently there have been multiple conflicting reports on the effect of H2S which could be largely attributed to the source and dose of H2S studied.
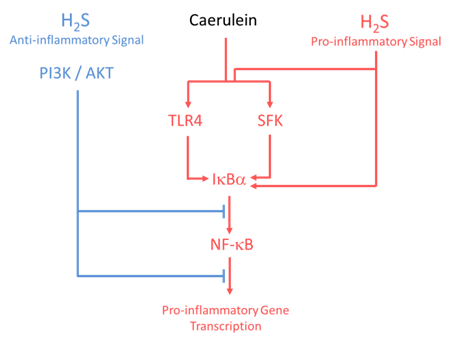
Figure 2. Based on our findings, this overview shows that H2S (5µM) induced (PI3K/AKT) anti-inflammatory signals down-regulate proinflammatory gene induction by abrogating caerulein-induced IkBα degradation and thus NF-kB nuclear translocation. On the contrary, H2S at 100µM concentration stimulates SFK, TLR4 phosphorylation, IkBα degradation and thus nuclear translocation of NF-kB to up-regulate proinflammatory gene induction
3. Tools for Study of H2S
a. Knockout mice and transgenic cell lines
- PPTA knockout mice of BALB/c background (11)
- CSE knockout mice of C57BL/6J background (58)
- CSE knockout mice of C57BL/6J background (19)
- CSE overexpressed HEK-293 cells (47)
b. CSE inhibitors
- D,L-propargylglycine, Compound ID: 95575 (1)
- β-cyano-L-alanine, Compound ID: 439742 (1)
c. CBS inhibitors
- Hydroxylamine, Compound ID: 787 (1)
- Aminooxyacetate, Compound ID 286 (1)
d. CBS activator
- S-adenosyl-L-methionine(AdoMet), Compound ID: 34756 (25)
e. H2S donor compounds
- NaHS (Compound ID: 28015)
- Na2S (Compound ID: 237873)
- SPRC (US Patent application – US 2009/0036534 A1
- GYY4137 (30)
f. PCR primers (24)
- Mouse Cystathionine-γ-lyase
Forward 5’ – ATG GAT GAA GTG TAT GGA GG -3’
Reverse 5′ -ACG AAG CCG ACT ATT GAG GT- 3’ - Mouse Cystathionine-β-synthase
Forward 5’ – ACT ACG ATG ACA CCG CCG AG – 3’
Reverse 5’ – AGT CCT TCC TGT GCG ATG AG – 3’
g. Antibodies
- Rabbit anti mouse CSE and CBS antibody for immunofluorescence staining in pancreatic tissue and western blotting of pancreatic islet cell protein extract (23).
h. Method of measuring H2S
To date, several methods of measuring sulfides in biological samples have been developed (50). Among the more commonly used methods are:
- Spectrophotometric method (Methylene Blue Assay)
- A more high-throughput method
- Sensitivity in the micromolar range
- Chromatography (Ion, Liquid and Gas)
- More specific as sulfide is separated before measurement
- Sensitivity depends on the method of sulfide binding and detection employed.
- Sulfide Specific Electrode
- Currently the most specific and sensitive method
- Allows for real-time measurement
- Very slow turn-over rate
4. References
- Abe K, Kimura H. The possible role of hydrogen sulfide as an endogenous neuromodulator. J Neurosci 16: 1066-1071,1996. PMID: 8558235
- Ali MY, Whiteman M, Low CM, Moore PK. Hydrogen sulphide reduces insulin secretion from HIT-T15 cells by a KATP channel-dependent pathway. J Endocrinol 195: 105-112, 2007. PMID: 17911402
- Andruski B, McCafferty DM, Ignacy T, Millen B, McDougall JJ. Leukocyte trafficking and pain behavioral responses to a hydrogen sulfide donor in acute monoarthritis. Am J Physiol Regul Integr Comp Physiol 295: R814-R820, 2008. PMID: 18667709
- Bao L, Vlcek C, Paces V, Kraus JP. Identification and tissue distribution of human cystathionine beta-synthase mRNA isoforms. Arch Biochem Biophys 350: 95-103,1998. PMID: 9466825
- Beauchamp RO Jr, Bus JS, Popp JA, Boreiko CJ, Andjelkovich DA. A critical review of the literature on hydrogen sulfide toxicity. Critical Reviews in Toxicology 13: 25-97, 1984. PMID: 6378532
- Bhatia M, Sidhapuriwala JN, Sparatore A, Moore PK. Treatment with H2S-releasing diclofenac protects mice against acute pancreatitis-associated lung injury. Shock 29: 84-88, 2008. PMID: 17621252
- Bhatia M, Sidhapuriwala JN, Ng SW, Tamizhselvi R, Moochhala SM. Pro-inflammatory effects of hydrogen sulphide on substance P in caerulein-induced acute pancreatitis. J Cell Mol Med 12: 580-590, 2008. PMID: 18419599
- Bhatia M, Wong FL, Fu D, Lau HY, Moochhala SM, Moore PK. Role of hydrogen sulfide in acute pancreatitis and associated lung injury. FASEB J 19: 623-625, 2005. PMID: 15671155
- Bian JS, Yong QC, Pan TT, Feng ZN, Ali MY, Zhou S, Moore PK. Role of hydrogen sulfide in the cardioprotection caused by ischemic preconditioning in the rat heart and cardiac myocytes. J Pharmacol Exp Ther 316: 670-678, 2005. PMID: 16204473
- Cao Y, Adhikari S, Ang AD, Moore PK, Bhatia M. Mechanism of induction of pancreatic acinar cell apoptosis by hydrogen sulfide. Am J Physiol Cell Physiol 291: C503-C510, 2006. PMID:16597918
- Cao YQ, Mantyh PW, Carlson EJ, Gillespie AM, Epstein CJ, Basbaum A. Primary afferent tachykinins are required to experience moderate to intense pain. Nature 392: 390-394, 1998. PMID: 9537322
- Dal-Secco D, Cunha TM, Freitas A, Alves-Filho JC, Souto FO, Fukada SY, Grespan R, Alencar NM, Neto AF, Rossi MA, Ferreira SH, Hothersall JS, Cunha FQ. Hydrogen sulfide augments neutrophil migration through enhancement of adhesion molecule expression and prevention of CXCR2 internalization: role of ATP-sensitive potassium channels. J Immunol 181: 4287-4298, 2008. PMID: 18768887
- Della Coletta Francescato H, Cunha FQ, Costa RS, Barbosa Júnior F, Boim MA, Arnoni CP, da Silva CG, Coimbra TM. Inhibition of hydrogen sulphide formation reduces cisplatin-induced renal damage. Nephrol Dial Transplant 26: 479-488, 2011. PMID: 20656754
- Dello Russo C, Tringali G, Ragazzoni E, Maggiano N, Menini E, Vairano M, Preziosi P, Navarra P. Evidence that hydrogen sulphide can modulate hypothalamo-pituitary-adrenal axis function: in vitro and in vivo studies in the rat. J Neuroendocrinol 12: 225-33, 2000. PMID: 10718918
- Distrutti E, Sediari L, Mencarelli A, Renga B, Orlandi S, Antonelli E, Roviezzo F, Morelli A, Cirino G, Wallace JL, Fiorucci S. Evidence that hydrogen sulfide exerts antinociceptive effects in the gastrointestinal tract by activating KATP channels. J Pharmacol Exp Ther 316: 325-335, 2006. PMID: 16192316
- Fukushima O, Nishimura S, Matsunami M, Aoki Y, Nishikawa H, Ishikura H, Kawabata A. Phosphorylation of ERK in the spinal dorsal horn following pancreatic pronociceptive stimuli with proteinase-activated receptor-2 agonists and hydrogen sulfide in rats: Evidence for involvement of distinct mechanisms. J Neurosci Res 88: 3198-3205, 2010. PMID: 20806405
- Goodwin LR, Francom D, Dieken FP, Taylor JD, Warenycia NW, Reiffenstein RJ, Dowling G. Determination of sulfide in brain tissue by gas dialysis/ion chromatography: post-mortem studies and two case reports. J Anal Toxicol 13:105-109, 1989. PMID: 2733387
- Hosoki R, Matsuki N, Kimura H. The possible role of hydrogen sulfide as an endogenous smooth muscle relaxant in synergy with nitric oxide. Biochem Biophys Res Commun 237: 527-531, 1997. PMID: 9299397
- Ishii I, Akahoshi N, Yamada H, Nakano S, Izumi T, Suematsu M. Cystathionine gamma-Lyase-deficient mice require dietary cysteine to protect against acute lethal myopathy and oxidative injury. J Biol Chem 285: 26358-26368, 2010. PMID: 20566639
- Ishii I, Akahoshi N, Yu XN, Kobayashi Y, Namekata K, Komaki G, Kimura H. Murine cystathionine gamma-lyase: complete cDNA and genomic sequences, promoter activity, tissue distribution and developmental expression. Biochem J 381: 113-123, 2004. PMID: 15038791
- Johansen D, Ytrehus K, Baxter GF. Exogenous hydrogen sulfide (H2S) protects against regional myocardial ischemia-reperfusion injury--Evidence for a role of K ATP channels. Basic Res Cardiol 101: 53-60, 2006. PMID: 16328106
- Kamoun P. Endogenous production of hydrogen sulphide in mammals. Amino Acids 26: 243-254, 2004. PMID: 15221504
- Kaneko Y, Kimura T, Taniguchi S, Souma M, Kojima Y, Kimura Y, Kimura H, Niki I. Glucose-induced production of hydrogen sulfide may protect the pancreatic beta-cells from apoptotic cell death by high glucose. FEBS Lett 583: 377-382, 2009. PMID: 19100738
- Kaneko Y, Kimura Y, Kimura H, Niki I. L-cysteine inhibits insulin release from the pancreatic beta-cell: possible involvement of metabolic production of hydrogen sulfide, a novel gasotransmitter. Diabetes 55: 1391-1397, 2006. PMID: 16644696
- Kery V, Bukovska G, Kraus JP. Transsulfuration depends on heme in addition to pyridoxal 5'-phosphate. Cystathionine beta-synthase is a heme protein. J Biol Chem 269: 25283-25288, 1994. PMID: 7929220
- Kimura Y, Kimura H. Hydrogen sulfide protects neurons from oxidative stress. FASEB J 18: 1165-1167, 2004. PMID: 15155563
- Laggner H, Muellner MK, Schreier S, Sturm B, Hermann M, Exner M, Gmeiner BM, Kapiotis S. Hydrogen sulphide: a novel physiological inhibitor of LDL atherogenic modification by HOCl. Free Radic Res 41: 741-747, 2007. PMID: 17577734
- Li L, Bhatia M, Zhu YZ, Zhu YC, Ramnath RD, Wang ZJ, Anuar FB, Whiteman M, Salto-Tellez M, Moore PK. Hydrogen sulfide is a novel mediator of lipopolysaccharide-induced inflammation in the mouse. FASEB J 19: 1196-1198, 2005. PMID: 15863703
- Li L, Rossoni G, Sparatore A, Lee LC, Del Soldato P, Moore PK. Anti-inflammatory and gastrointestinal effects of a novel diclofenac derivative. Free Radic Biol Med 42: 706-719, 2007. PMID: 17291994
- Li L, Salto-Tellez M, Tan CH, Whiteman M, Moore PK. GYY4137, a novel hydrogen sulfide-releasing molecule, protects against endotoxic shock in the rat. Free Radic Biol Med 47: 103-113, 2009. PMID: 19375498
- Matsunami M, Tarui T, Mitani K, Nagasawa K, Fukushima O, Okubo K, Yoshida S, Takemura M, Kawabata A. Luminal hydrogen sulfide plays a pronociceptive role in mouse colon. Gut 58: 751-761, 2009. PMID: 18852258
- Mitsuhashi H, Yamashita S, Ikeuchi H, Kuroiwa T, Kaneko Y, Hiromura K, Ueki K, Nojima Y. Oxidative stress-dependent conversion of hydrogen sulfide to sulfite by activated neutrophils. Shock 24: 529-534, 2005. PMID: 16317383
- Mok YY, Moore PK. Hydrogen sulphide is pro-inflammatory in haemorrhagic shock. Inflam Res 57: 512-518, 2008. PMID: 19109743
- Moore PK, Bhatia M, Moochhala S. Hydrogen sulfide: from smell of the past to the mediator of the future. Trends Pharmacol Sci 24: 609-611, 2003. PMID: 14654297
- Nishimura S, Fukushima O, Ishikura H, Takahashi T, Matsunami M, Tsujiuchi T, Sekiguchi F, Naruse M, Kamanaka Y, Kawabata A. Hydrogen sulfide as a novel mediator for pancreatic pain in rodents. Gut 58: 762-770, 2009. PMID: 19201768
- Ogasawara Y, Isoda S, Tanabe S. Tissue and subcellular distribution of bound and acid labile sulfur, and the enzymic capacity for sulphide production in the rat. Biol Pharm Bull 17: 1535-1542, 1994. PMID: 7735193
- Prentki M, Matschinsky FM. Ca2+, cAMP, and phospholipid-derived messengers in coupling mechanisms of insulin secretion. Physiol Rev 67: 1185-1248, 1987. PMID: 2825225
- Quéré I, Paul V, Rouillac C, Janbon C, London J, Demaille J, Kamoun P, Dufier JL, Abitbol M, Chassé JF. Spatial and temporal expression of the cystathionine beta-synthase gene during early human development. Biochem Biophys Res Commun 254: 127-137, 1999. PMID: 9920745
- Sidhapuriwala JN, Ng SW, Bhatia M. Effects of hydrogen sulfide on inflammation in caerulein-induced acute pancreatitis. J Inflamm (Lond) 6: 35, 2009. PMID: 20040116
- Sivarajah A, Collino M, Yasin M, Benetti E, Gallicchio M, Mazzon E, Cuzzocrea S, Fantozzi R, Thiemermann C. Anti-apoptotic and anti-inflammatory effects of hydrogen sulfide in a rat model of regional myocardial I/R. Shock 31: 267-274, 2009. PMID: 18636044
- Sodha NR, Clements RT, Feng J, Liu Y, Bianchi C, Horvath EM, Szabo C, Stahl GL, Sellke FW. Hydrogen sulfide therapy attenuates the inflammatory response in a porcine model of myocardial ischemia/reperfusion injury. J Thorac Cardiovasc Surg 138: 977-984, 2009. PMID: 19660398
- Stipanuk MH, Beck PW. Characterization of the enzymic capacity for cysteine desulphydration in the liver and kidney of the rat. Biochem J 206: 267-277, 1982. PMID: 7150244
- Takahashi T, Aoki Y, Okubo K, Maeda Y, Sekiguchi F, Mitani K, Nishikawa H, Kawabata A. Upregulation of Ca(v)3.2 T-type calcium channels targeted by endogenous hydrogen sulfide contributes to maintenance of neuropathic pain. Pain 150: 183-191, 2010. PMID: 20546998
- Tamizhselvi R, Koh YH, Sun J, Zhang H, Bhatia M. Hydrogen sulfide induces ICAM-1 expression and neutrophil adhesion to caerulein-treated pancreatic acinar cells through NF-kappaB and Src-family kinases pathway. Exp Cell Res 316: 1625-1636, 2010. PMID: 20211170
- Tamizhselvi R, Moore PK, Bhatia M. Inhibition of hydrogen sulfide synthesis attenuates chemokine production and protects mice against acute pancreatitis and associated lung injury. Pancreas 36: e24-e31, 2008. PMID: 18437075
- Tamizhselvi R, Moore PK, Bhatia M. Hydrogen sulfide acts as a mediator of inflammation in acute pancreatitis: in vitro studies using isolated mouse pancreatic acinar cells. J Cell Mol Med 11: 315-326, 2007. PMID: 17488480
- Tamizhselvi R, Shrivastava P, Koh YH, Zhang H, Bhatia M. Preprotachykinin-a gene deletion regulates hydrogen sulfide-induced toll-like receptor 4 signaling pathway in cerulein-treated pancreatic acinar cells. Pancreas 40: 444-452, 2011. PMID: 21289528
- Tamizhselvi R, Sun J, Koh YH, Bhatia M. Effect of hydrogen sulfide on the phosphatidylinositol 3-kinase-protein kinase B pathway and on caerulein-induced cytokine production in isolated mouse pancreatic acinar cells. J Pharmacol Exp Ther 329: 1166-1177, 2009. PMID: 19258518
- Taniguchi S, Kang L, Kimura T, Niki I. Hydrogen sulphide protects mouse pancreatic beta-cells from cell death induced by oxidative stress, but not by endoplasmic reticulum stress. Br J Pharmacol 162: 1171-1178, 2011. PMID: 21091646
- Ubuka T. Assay methods and biological roles of labile sulfur in animal tissues. J Chromatogr B Analyt Technol Biomed Life Sci 781: 227-249, 2002. PMID: 12450661
- Wallace JL, Dicay M, McKnight W, Martin GR. Hydrogen sulfide enhances ulcer healing in rats. FASEB J 21: 4070-4076, 2007. PMID: 17634391
- Wallace JL, Vong L, McKnight W, Dicay M, Martin GR. Endogenous and exogenous hydrogen sulfide promotes resolution of colitis in rats. Gastroenterology 137: 569-578, 2009. PMID: 19375422
- Wang R. Two's company, three's a crowd: can H2S be the third endogenous gaseous transmitter?. FASEB J 16: 1792-1798, 2002. PMID: 12409322
- Whiteman M, Armstrong JS, Chu SH, Jia-Ling S, Wong BS, Cheung NS, Halliwell B, Moore PK. The novel neuromodulator hydrogen sulfide: an endogenous peroxynitrite 'scavenger'?. J Neurochem 90: 765-768, 2004. PMID: 15255956
- Whiteman M, Cheung NS, Zhu YZ, Chu SH, Siau JL, Wong BS, Armstrong JS, Moore PK. Hydrogen sulphide: a novel inhibitor of hypochlorous acid-mediated oxidative damage in the brain?. Biochem Biophys Res Commun 326: 794-798, 2005. PMID: 15607739
- World Health Organization. Hydrogen Sulfide: Human Health Aspects. Concise International Chemical Assessment Document 53. Stuttgart, Germany, 2003.
- Wu L, Yang W, Jia X, Yang G, Duridanova D, Cao K, Wang R. Pancreatic islet overproduction of H2S and suppressed insulin release in Zucker diabetic rats. Lab Invest 89: 59-67, 2009. PMID: 19002107
- Yang G, Wu L, Jiang B, Yang W, Qi J, Cao K, Meng Q, Mustafa AK, Mu W, Zhang S, Snyder SH, Wang R. H2S as a physiologic vasorelaxant: hypertension in mice with deletion of cystathionine gamma-lyase. Science 322: 587-590, 2008. PMID: 18948540
- Yang G, Yang W, Wu L, Wang R. H2S, endoplasmic reticulum stress, and apoptosis of insulin-secreting beta cells. J Biol Chem 282: 16567-16576, 2007. PMID: 17430888
- Yang W, Yang G, Jia X, Wu L, Wang R. Activation of KATP channels by H2S in rat insulin-secreting cells and the underlying mechanisms. J Physiol 569: 519-531, 2005. PMID: 16179362
- Yao LL, Huang XW, Wang YG, Cao YX, Zhang CC, Zhu YC. Hydrogen sulfide protects cardiomyocytes from hypoxia/reoxygenation-induced apoptosis by preventing GSK-3beta-dependent opening of mPTP. Am J Physiol Heart Circ Physiol 298: H1310-H1319, 2010. PMID: 20154265
- Yong QC, Lee SW, Foo CS, Neo KL, Chen X, Bian JS. Endogenous hydrogen sulphide mediates the cardioprotection induced by ischemic postconditioning. Am J Physiol Heart Circ Physiol 295: H1330-H1340, 2008. PMID: 18660450
- Yusuf M, Kwong Huat BT, Hsu A, Whiteman M, Bhatia M, Moore PK. Streptozotocin-induced diabetes in the rat is associated with enhanced tissue hydrogen sulfide biosynthesis. Biochem Biophys Res Commun 333: 1146-1152, 2005. PMID: 15967410
- Zanardo RC, Brancaleone V, Distrutti E, Fiorucci S, Cirino G, Wallace JL. Hydrogen sulfide is an endogenous modulator of leukocyte-mediated inflammation. FASEB J 20: 2118-2120, 2006. PMID: 16912151
- Zhang H, Zhi L, Moochhala S, Moore PK, Bhatia M. Hydrogen sulfide acts as an inflammatory mediator in cecal ligation and puncture-induced sepsis in mice by upregulating the production of cytokines and chemokines via NF-kappaB. Am J Physiol Lung Cell Mol Physiol 292: L960-L971, 2007. PMID: 17209138
- Zhang J, Sio SW, Moochhala S, Bhatia M. Role of hydrogen sulfide in severe burn injury-induced inflammation in mice. Mol Med 16: 417-424, 2010. PMID: 20440442
- Zhao W, Zhang J, Lu Y Wang R. The vasorelaxant effect of H2S as a novel endogenous KATP channel opener. EMBO J 20: 6008-6016, 2001. PMID: 11689441
- Zhi L, Ang AD, Zhang H, Moore PK, Bhatia M. Hydrogen sulfide induces the synthesis of proinflammatory cytokines in human monocyte cell line U937 via the ERK-NF-kappaB pathway. J Leukoc Biol 81: 1322-1332, 2007. PMID: 17289797